New computing allocation will help to predict detonation behavior
A new collaboration between the College of Engineering and the Rosen Center for Advanced Computing (RCAC) will simulate energetic materials for the Department of Defense, modeling them at multiple scales to help predict and prevent unwanted detonation.
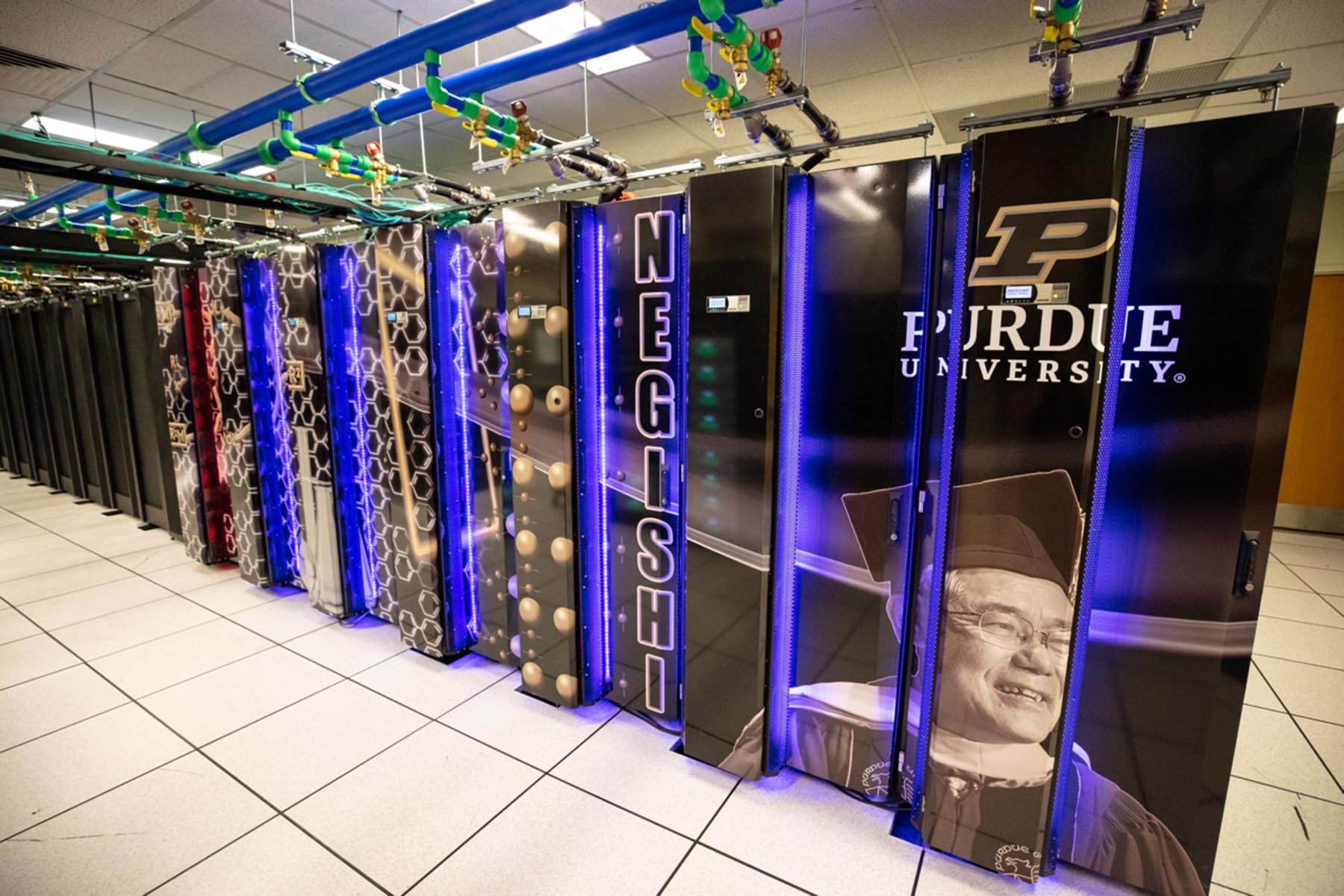
“The detonation of energetic materials is a complex process that happens at extremely fast time scales,” said Marisol Koslowski, professor of mechanical engineering and co-principal investigator of the project. “But it also happens across multiple length scales as well — from microscopic grains all the way down to the molecular level, and sometimes even smaller.”
Koslowski’s group specializes in modeling materials nanometer-sized and larger. Co-principal investigator Alejandro Strachan, Reilly Professor of Materials Engineering, specializes in modeling materials nanometer-sized and smaller. Both scales are needed to fully understand how detonations happen; however, modeling at these two scales requires very different techniques.
“Atomic simulations are very powerful at capturing the mechanochemical response of these materials, but they are very computationally intensive,” Koslowski said. “Once we get to my scale, where the millimeters matter, resolving all those atoms and molecules in a computer simulation is not feasible.”
Their goal is to build a bridge between the two scales, using artificial intelligence. By working with the results of Strachan’s molecular dynamics simulations, they will develop models using machine learning, and apply them to the larger particles using finite element analysis. This will enable the team to build a more complete picture of the entire detonation process more quickly and more efficiently.
“We will use machine learning to infer properties of the microstructure from the molecular structure,” Koslowski said. “If I’m studying a crack in the material, or an interface between two different materials, building my models from the molecular dynamics models will help the simulations be more accurate and calculated more quickly.”
Super computers
To accomplish this, the team requires significant computing power. That’s why they collaborated with Purdue’s Rosen Center for Advanced Computing (RCAC) to purchase nodes in the Gilbreth and Negishi community clusters, two powerful supercomputers. Negishi, Purdue’s newest community cluster, is optimized for traditional, tightly-coupled science and engineering applications, while Gilbreth is optimized for communities running GPU intensive applications and was built to support RCAC’s ongoing investment in researchers doing AI and machine learning work.
RCAC’s Community Cluster Program makes more computing power available for Purdue researchers than faculty and campus units could individually afford, and provides this crucial service at the lowest cost to the institution. RCAC installs, administers and maintains the community clusters, providing reliability, security, software installation and expert user support. Researchers in the community cluster program always have dedicated access to the nodes they purchase, and can potentially access much more if they need it, because computer nodes are shared among community cluster partners when idle.
The purchase is possible thanks to a grant from the Defense University Research Instrumentation Program (DURIP), a Department of Defense program which supports universities purchasing new equipment to conduct research relevant to national defense. In this case, the DURIP to purchase the new Community Cluster nodes includes $520,800 from the Air Force Office of Scientific Research (AFOSR), with an additional $250,000 for salaries, travel, and publication.
Purdue University is well-known for its research in energetic materials, and Koslowski hopes that this computing collaboration will advance the science even further. “It’s a difficult field,” she said. “Modeling most materials is relatively easy, but everything in energetic materials is multi-physics: mechanics, chemistry, thermodynamics. The chemistry of a material dictates its mechanical structure, the structure induces heat, the heat induces pressure. Multiple mechanisms cause detonation, which in turn changes all those properties as it occurs.”
She continued: “Right now, most energetic materials research is experimental — researchers detonate these materials, and then observe what happens. We want our models to be so robust that you could look at the microstructure before detonation, and be able to predict exactly when and where shockwaves will take place. That will help us prevent unwanted detonation, and make the use of energetic materials safer and more predictable than ever before.”
Writer: Jared Pike, jaredpike@purdue.edu, 765-496-0374
Source: Marisol Koslowski, marisol@purdue.edu
Alejandro Strachan, strachan@purdue.edu