Bursting the computational bubble of liquid-vapor phase change
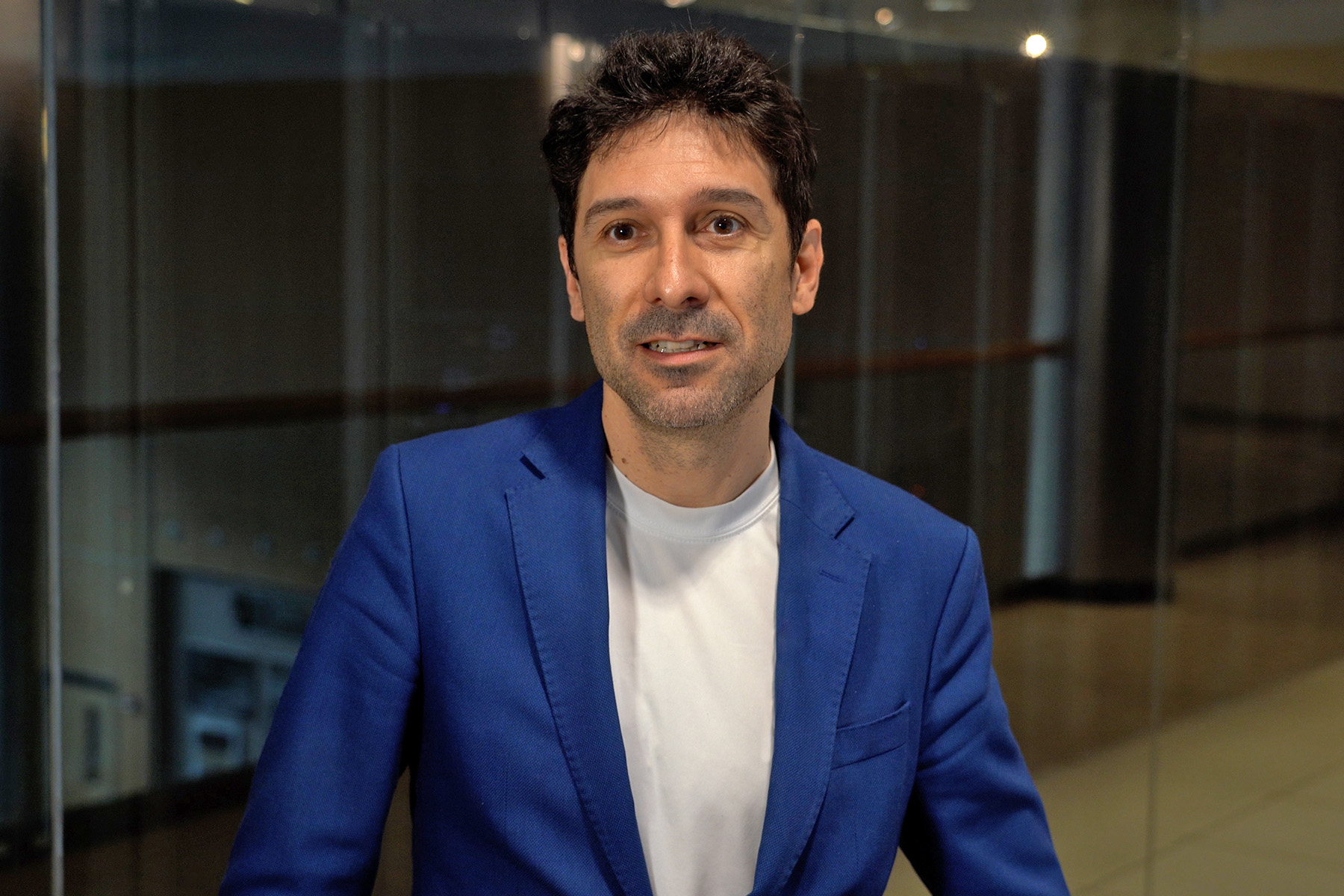
“Predicting anything about a flow that involves transformation between liquid and vapor is extremely difficult,” said Hector Gomez, Professor of Mechanical Engineering. “Most modeling of the liquid-to-vapor transformation in flowing fluids is based on empiricism. Those models are built around specific thermodynamic or flow conditions; so if any of those conditions change, the model may not work. What we wanted to do was take existing first-principle models, and find a computational method to use them in engineering practice, without requiring enormous amounts of computing power.”
Their research has been published in Science Advances.
In attempting to model liquid-to-vapor transition, researchers have traditionally relied upon van der Waals’ thermodynamic theories related to liquid-vapor phase change. Based on those theories, fluid dynamics researchers use a system called Navier-Stokes-Korteweg equations to model such complex flows. But unfortunately, these models have only been utilized at the nanoscale, limiting their usefulness in real-world applications.
“Simulating these flows involves resolving the interface between liquid and vapor,” said Tianyi Hu, Ph.D. student in Gomez’ lab. “These are nanoscale density variations, which need to be resolved by the computational method. But we found a way to systematically enlarge the interface, without altering any other property of the system, so it becomes resolvable at a much larger scale.”
The team calls their approach “Direct van der Waals Simulation,” because it relies solely on the fundamental principles put forward under van der Waals’ thermodynamic theory. It doesn’t rely on empirical models, which must change based on the physical conditions involved.
The second breakthrough involves a stabilization algorithm that reduces the computational complexity required to solve liquid-vapor phase transformations in complicated flows. “At the mesoscale, predicting these flows becomes much more chaotic,” said Hu. “But with our algorithm, it becomes solvable, and usable in engineering practice.”
They confirmed their theories by running simulations of cavitating flow over a cylinder and a wedge. Using their Direct van der Waals Simulation method, they achieved the same results that were previously observed experimentally.
“Interestingly, there was not a lot of trial-and-error,” said Gomez. “The model is so good, because it’s based on fundamental first principles of liquid and vapor that are well understood. The breakthrough was being able to develop the algorithms to use those first principles directly.”
Gomez looks forward to seeing these complex fluid modeling techniques applied in several fields. “Liquid-vapor phase change is being explored for semiconductors, to remove massive amounts of heat from computer processors,” said Gomez. “We plan to work with the Cooling Technologies Research Center to model how these flows behave, and how they can be used to cool microelectronics. Cavitation is also a part of national defense, modeling how the forces of bursting bubbles cause real damage to submarines and other ships. And it also applies to biomedicine, determining how cavitation affects the brain and other parts of the body.”
This work is funded partially by the U.S. Department of Defense (Award No. FA9550-20-1-0165) and partially by National Science Foundation (Award No. 1805817).
Writer: Jared Pike, jaredpike@purdue.edu, 765-496-0374
Source: Hector Gomez, hectorgomez@purdue.edu
Direct van der Waals simulation (DVS) of phase-transforming fluids
Tianyi Hu, Hao Wang and Hector Gomez
https://www.science.org/doi/10.1126/sciadv.adg3007
ABSTRACT: We present the method of Direct van der Waals simulation (DVS) to study computationally flows with liquid-vapor phase transformations. Our approach is based on a novel discretization of the Navier-Stokes-Korteweg equations, that couple flow dynamics with van der Waals’ non-equilibrium thermodynamic theory of phase transformations, and opens an opportunity for first-principles simulation of a wide range of boiling and cavitating flows. The proposed algorithm enables unprecedented simulations of the Navier-Stokes-Korteweg equations involving cavitating flows at strongly under-critical conditions and O(105) Reynolds number. The proposed technique provides a pathway for fundamental understanding of phase-transforming flows with multiple applications in science, engineering, and medicine.