Using microfluidics to manufacture hydrophobic drugs
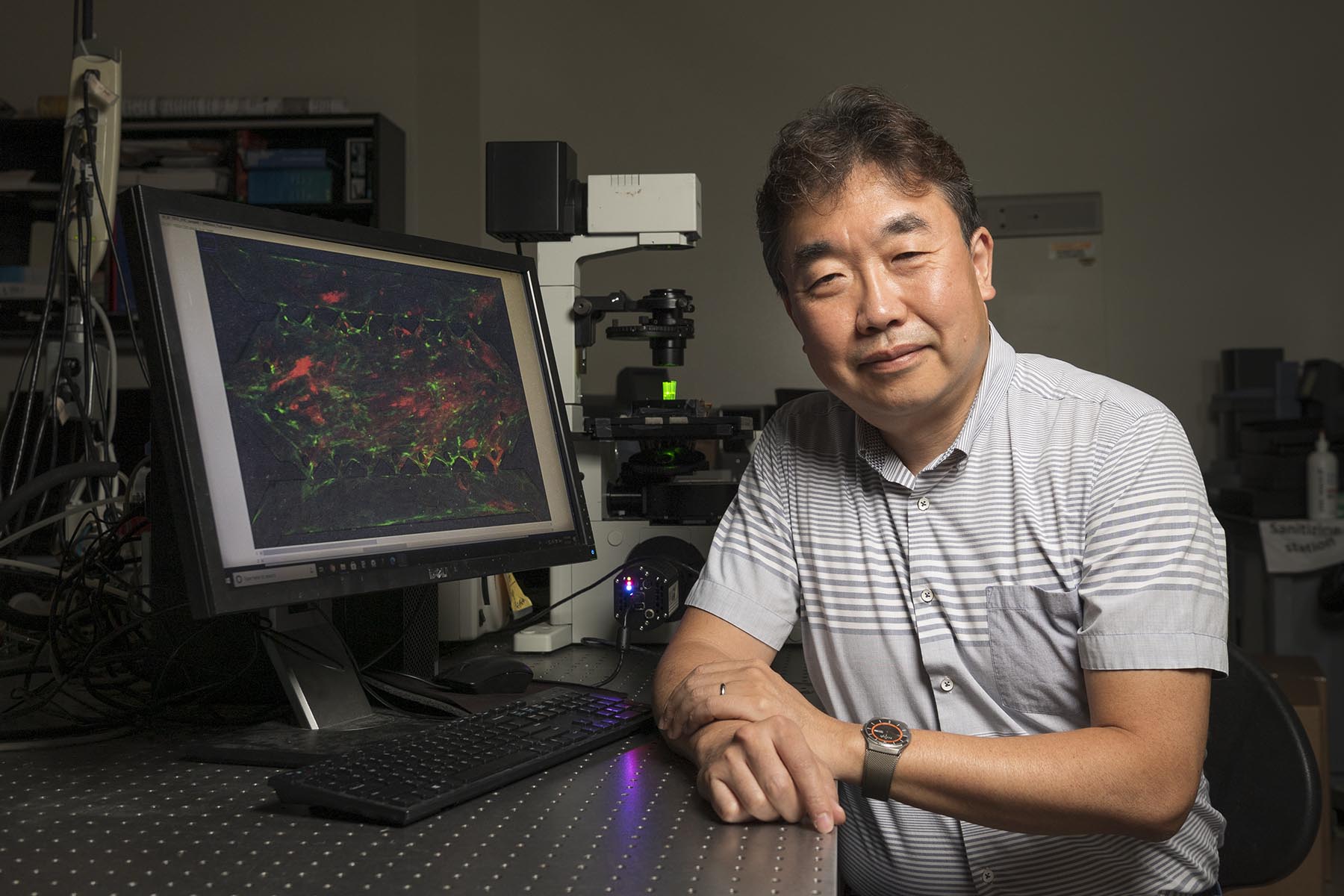
The project began as a collaboration between Bumsoo Han, professor of mechanical engineering, and Yoon Yeo, Lillian Barboul Thomas Professor of Industrial and Physical Pharmacy and Biomedical Engineering. Yeo’s pharmacy researchers recognized the promise of this manufacturing method for hydrophobic drugs, but had difficulties synthesizing specific types of nanoparticles. “They turned to us because we had lots of experience using microfluidics to control nanoparticles, especially when it comes to cancer treaments,” said Yingnan Shen, a postdoctoral researcher with Han, and lead author on the published paper.
But Shen soon realized he had a lot to learn about how pharmaceuticals are made. “There are two basic methods to make drugs: top-to-bottom, or bottom-to-top,” he said. “Top-to-bottom involves taking a substance, like a particle or powder, and grinding it into smaller particles. This can be done at scale, which is how most drugs are made today. But that’s not practical when hydrophobic nanoparticles are involved. We have to develop new bottom-to-top methods, using fluid mechanics to combine the drug into nanocapsules with specific properties.”
Their research has been published in the Journal of Controlled Release.
70% to 80% of the compounds developed today for pharmaceuticals are hydrophobic, meaning they have poor solubility in water. Unlike traditional pills you take into your body (where the molecules break down in your stomach), hydrophobic substances require some other method to get the medicine absorbed into the body.
One approach is to coat the particles in a thin shell of tannic acid and iron chloride, biocompatible substances that are pH-responsive and can dissolve in water to release the nanoparticles in a customized and controlled manner – from hours to even days. This approach is particularly vital when cancerous tumors are involved, and the controlled release of a drug is important to the treatment.
So then the question became: how do you get these three ingredients (tannic acid, iron chloride, and newly-developed nanoparticle-based drugs) to combine into this coated capsule? And how do you do it at scale, where it becomes practical to manufacture as a pharmaceutical? To answer these questions, Bumsoo Han’s team turned to microfluidics: tiny devices with microfluidic channels, which they have used to mimic environments in the body, developing new methods of diagnosing and treating cancer.
“There are two mechanisms we need to study in order to make this work,” said Shen. “The first is fluid dynamics: how can we control those three ingredients mixing together? The second is nanoprecipitation: how do the drug molecules form a nucleus from which these particles grow? We need to optimize those conditions for different drugs.”
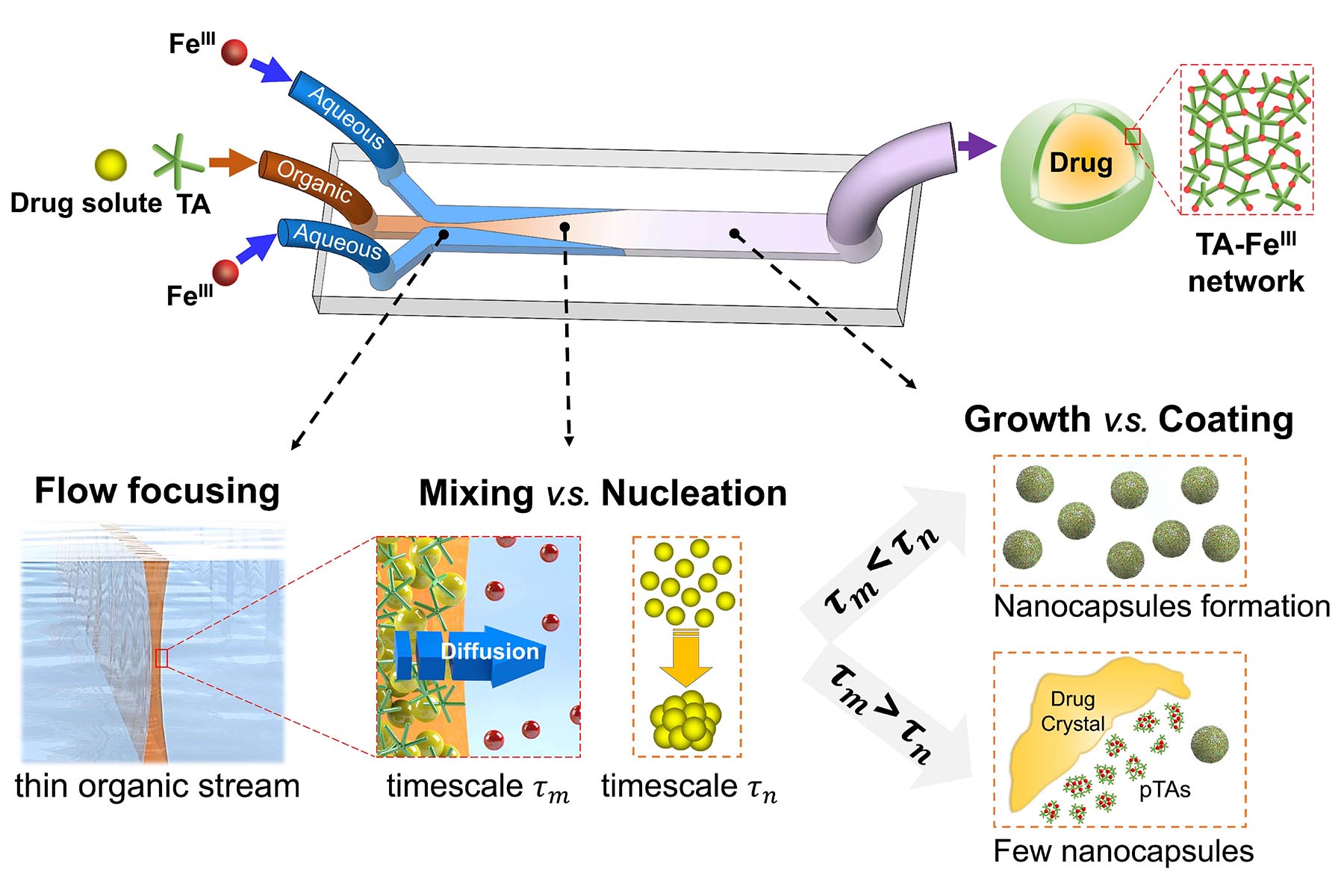
They tested the process using three hydrophobic drugs: paclitaxel, curcumin, and vitamin D; these are well-known to cancer researchers, and also have different levels of solubility in water. Using their microfluidic devices, they created coated nanoparticles by mixing the drugs with tannic acid and iron chloride. They then measured the resulting nanoparticles using electron microscopy, infrared imagery, and other numerical methods. The resulting particles had a promising concentration of the drug, at the correct size, with a throughput ideal for drug delivery. The team also measured the release kinetics, cellular uptake, and cytotoxicity.
“It took us about two years to arrive at the ideal process,” said Shen. “We did a lot of simulation and calculation first. And then we started making nanoparticles of vitamin D, which was the hardest one – but also the one that pharmacy researchers are most eager to use. Once we achieved that process, the other drugs became easier to make.”
The team has performed the foundational work for making drugs at this level; the next challenge is how to scale up the process. “Making drugs with this nanostructure coating has significant benefits,” said Shen. “It is non-toxic, can load effective levels of the drug, and can be released in a controlled manner. Now we want to develop this recipe further, and get these drugs into a form that can benefit people.”
Writer: Jared Pike, jaredpike@purdue.edu, 765-496-0374
Source: Bumsoo Han, bumsoo@purdue.edu
A timescale-guided microfluidic synthesis of tannic acid-FeIII network nanocapsules of hydrophobic drugs
Yingnan Shen, Simseok A. Yuk, Soonbum Kwon, Hassan Tamam, Yoon Yeo, Bumsoo Han
https://doi.org/10.1016/j.jconrel.2023.04.024
ABSTRACT: Many drugs are poorly water-soluble and suffer from low bioavailability. Metal-phenolic network (MPN), a hydrophilic thin layer such as tannic acid (TA)-FeIII network, has been recently used to encapsulate hydrophobic drugs to improve their bioavailability. However, it remains challenging to synthesize nanocapsules of a wide variety of hydrophobic drugs and to scale up the production in a continuous manner. Here, we present a microfluidic synthesis method to continuously produce TA-FeIII network nanocapsules of hydrophobic drugs. We hypothesize that nanocapsules can continuously be formed only when the microfluidic mixing timescale is shorter than the drug's nucleation timescale. The hypothesis was tested on three hydrophobic drugs - paclitaxel, curcumin, and vitamin D with varying solubility and nucleation timescale. The proposed mechanism was validated by successfully predicting the synthesis outcomes. The microfluidically-synthesized nanocapsules had well-controlled sizes of 100–200 nm, high drug loadings of 40–70%, and a throughput of up to 70 mg hr−1 per channel. The release kinetics, cellular uptake, and cytotoxicity were further evaluated. The effect of coating constituents on nanocapsule properties were characterized. Fe content of nanocapsules was reported. The stability of nanocapsules at different temperatures and pHs were also tested. The results suggest that the present method can provide a quantitative guideline to predictively design a continuous synthesis scheme for hydrophobic drug encapsulation via MPN nanocapsules with scaled-up capability.