Purdue researchers tame the interplay of light and matter to build a 'playground' for novel physics
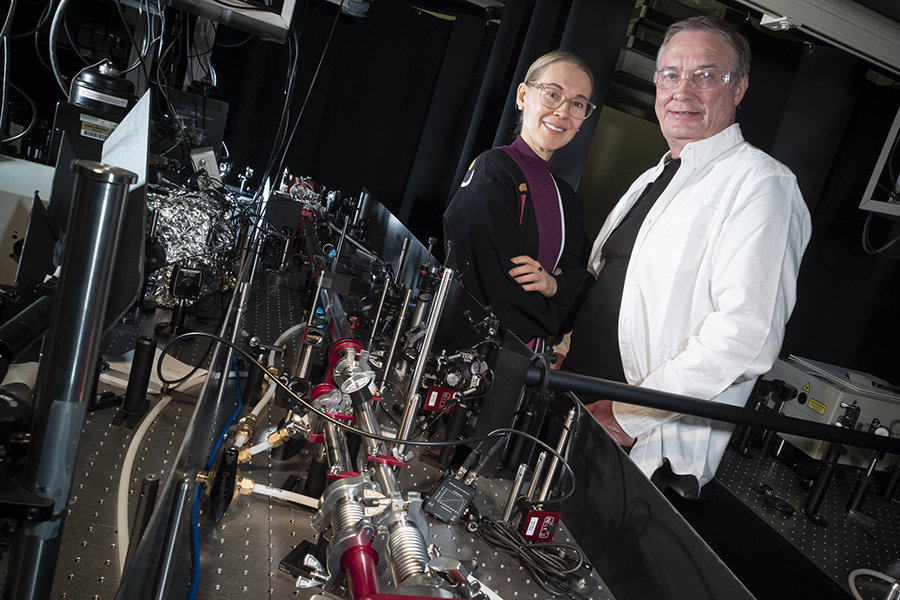
There are few things more essential to everyday technologies as we know them than the interplay between light and matter and the photons and electrons that constitute each. After all, this is how we see the world, as light is reflected or absorbed by different materials. The technology use cases of manipulating and exploiting these natural phenomena for human betterment are legion: lasers, solar cells, optical sensing, microscopy and communications, and nanophotonics, among others.
In a collaborative effort, Purdue University researchers have just made an impactful advancement in this field, publishing their findings in the journal Scientific Advances in a paper titled “Electronic Confinement-Induced Plasmonic Breakdown in Metals.”
"We have made great strides toward controlling the fundamental properties of electrons: how they behave on their own, and how they interact with light in a new class of atomically thin materials. This is where all optoelectronic devices for information technologies and sensing and imaging stem from,” said Alexandra (Sasha) Boltasseva, one of the paper’s authors, and the Ron and Dotty Garvin Tonjes Distinguished Professor in the Elmore Family School of Electrical and Computer Engineering.
Added Vladimir Shalaev, another of the paper’s authors, and the Bob and Anne Burnett Distinguished Professor of Electrical and Computer Engineering, “This advance may enable new applications for ultrathin metallic materials with thicknesses of only a few atomic layers that provide unprecedented tailorability in optical and electronic response, paving the way for novel optical and optoelectronic devices.”
The researchers are working with the so-called plasmonic materials, which have metallic properties where you have lots of free electrons, and light interacts with the collective oscillations of these free electrons called plasmons. Light interacts strongly with these “clouds” of free electrons in a hybrid system that is not exactly electrons nor exactly light. This strong interaction enables very high field localization, allowing one to focus light down to a nanoscale and support a system that is extremely sensitive to the environment — critical for high-sensitivity sensors. This also enables nanoimaging systems that require the fabrication of ultra-compact optical structures.
“This may be the best way to enable nanophotonics, by controlling light on a nanoscale,” said Shalaev.
The researchers have been exploring different materials that promote interaction of light with collective electron oscillations as well as control of the interactions between electrons. They work with what they called “transdimensional” materials: not truly 2D, because they have several atomic layers, nor exactly 3D thin films, which have many layers and act as a system with bulk material properties.
“We were interested to see how much plasmonic properties — the collective oscillation of electrons in a material — change in these ultra-thin films, where electrons start to move in a different way because they are now compressed in the thin structure,” said Boltasseva, who is also workforce development lead at the Quantum Science Center and a member of the Purdue Quantum Science and Engineering Institute. “This ‘quantum confinement’ influences the movement of electrons and the way they couple with light. We discovered that this material is highly tailorable, depending upon how you alter parameters such as thickness, strain or stress on the lattice, optical excitation, and so forth.”
The breakthrough for physics and engineering is that the researchers have discovered what is an extraordinary opportunity to work on materials with extremely high tunability. As they decreased the thickness of the material — they are experimenting with hafnium nitride — it became less metallic, and the electrons were more confined, not as mobile as before.
“At some point, the electrons are suddenly not moving and interacting with light, as if they’re frozen in some kind of lattice. That’s what we call plasmonic breakdown,” Boltasseva said.
What they have created is a single platform that supports vastly different behaviors for electrons. That enhanced tunability creates more options for a wide variety of devices. You can even have multiple functionalities in the same device; in principle, for example, you could combine a material that is a conductor in one state and an insulator in another state.
“It’s the advancement in fundamental physics from which new applications can often come,” said Shalaev. “We have built an important ‘playground’ in which one can demonstrate new fundamental phenomena.”
Added Boltasseva, “It’s important to emphasize that this new playground is much easier to use. It’s just a few atomic layers thick, and you can apply differing external stimuli for different optical responses, for everything from sensors to light modulators to wearables.”
The researchers believe that because this platform can be employed for studying the fundamentals of new physics, it could be a bigger discovery than some particular single application that may arise from their research innovation.
“Having a powerful theoretical model is much more important than just solving a particular problem, because that model can be applied to many problems. Similarly, having a new material platform that could be employed to study new fundamental phenomena is more important than one demonstrated application,” Shalaev said. Boltasseva seconds her co-researcher’s thought: “Physicists and engineers need new platforms in order for them to study new effects.”
The researchers emphasize that the transdimensional materials they introduced can enable a powerful and easy-to-use platform to study new physical phenomena. “If you look at other systems, you can observe some very interesting effects, but only at extreme conditions such as extremely low temperatures and high magnetic fields,” said Boltasseva. “Imagine that you would have these unusual properties at room temperature on a simple device that you could wear, place in a car or plane, or a host of other use cases.”
Like all great science, the research “stands on the shoulders of giants,” in the words of Sir Isaac Newton. The Purdue researchers cite several in their paper: the Hungarian-American physicist Eugene Wigner and his work around electron crystallization; the German physicist Paul Drude, who proposed a model to explain the transport properties of electrons in material; and the French physicist Charles-Augustin Coulomb, after whom the coulomb, a unit of electrical charge, is named.
They give credit in their research to colleagues Bivas Saha, associate professor at the International Center for Materials Science and Chemistry at the Jawaharlal Nehru Center for Advanced Scientific Research, who led experimental studies in this project and previously worked at Purdue at the Birck Nanotechnology Center on this transdimensional material; and Igor Bondarev, professor of Mathematics and Physics at North Carolina State University, who developed theory to uncover the fundamental physics behind the observed experimental results. Other authors include Prasanna Das, Sourav Rudra, Dheemahi Rao, Souvik Banerjee, and Ashalatha Indiradevi, all from Jawaharlal Nehru Centre for Advanced Scientific Research, and Kamalasanan Pillai and Magnus Garbrecht, from the University of Sydney.
“We seem to be positioned to observe exotic fundamental physics phenomena in a relatively simple material platform that has never been available before,” Shalaev said.
Quantum research is a pillar of Purdue Computes, a strategic university initiative to further scale Purdue’s research and educational excellence.