No more magnets: How an electrically driven, linear optical isolator on-a-chip opens door to new applications
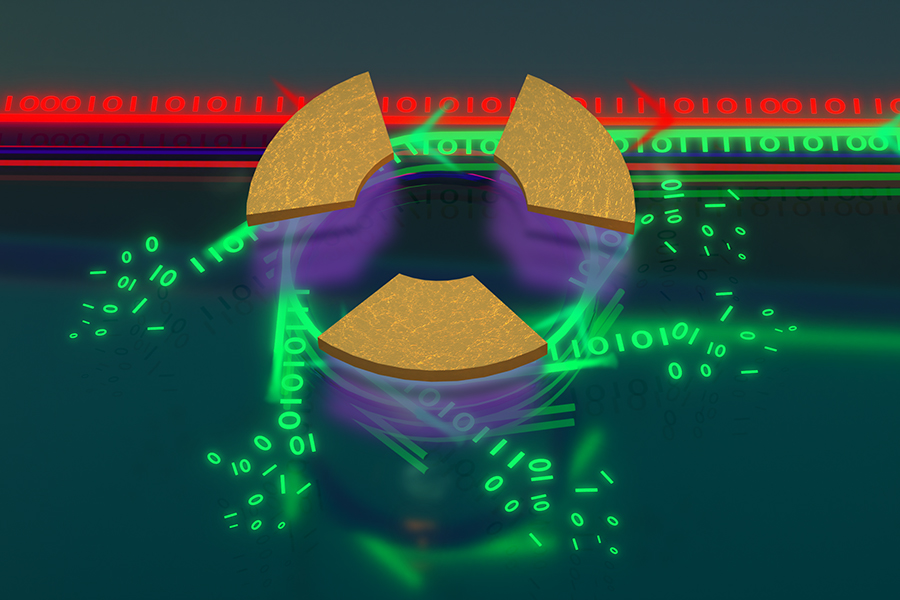
Traditional computers are fast, but nothing like the speed of light-at 186,000 miles per second, you can crunch numbers awfully quick. Thatâs a big reason why optical photonics are being used for photonic integrated circuits (PICs) to greatly increase bandwidth and speed.
“Integrated photonics—using light, and photons, instead of traditional electronics and electrons—has enabled chip-scale, compact optical systems with portable weight and low power consumption,” said Sunil Bhave, professor of electrical and computer engineering at The Elmore Family School of Electrical and Computer Engineering. “This has already been translated from tabletop research to commercial products—like chip-scale atomic clocks, light detection and ranging (LiDAR) systems for autonomous vehicles, photonic quantum computing that operates at room temperature, and on-chip spectroscopy for medical diagnosis, among others.”
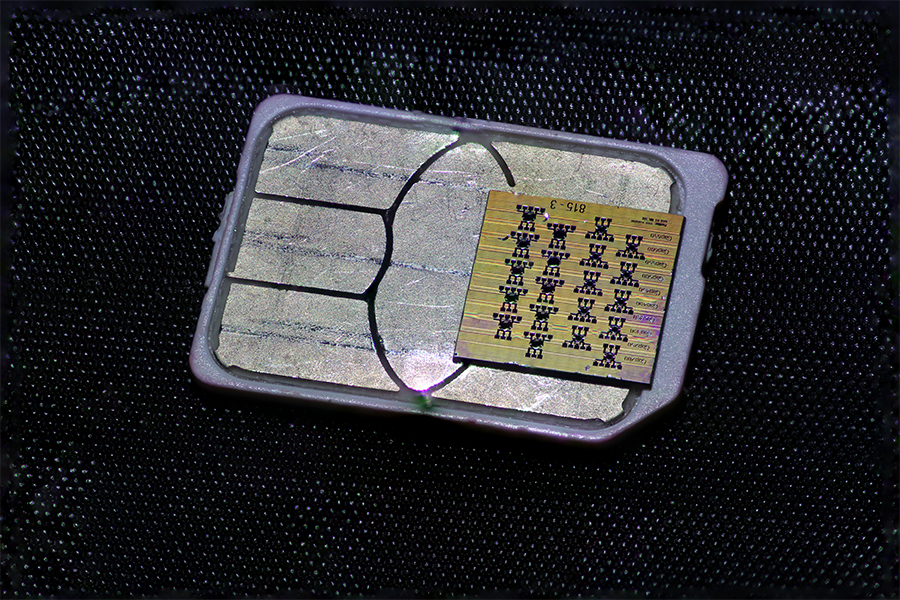
But the integration of on-chip optical gain elements—crucial to the laser’s ability to increase optical power—requires increased optical isolation, to protect the lasers from on-chip reflections, where the light can be reflected back onto the optical source and damage the equipment.
In traditional optics, magnetic materials and external bias magnetic fields are used to achieve this optical isolation through the magneto-optical phenomenon known as the Faraday effect, discovered by English scientist Michael Faraday in 1845. “However, the magnetic materials are not compatible with current semiconductor foundry processes—crucial for commercial manufacture at scale—and electrically driven optical isolators are highly desired,” Bhave says.
Bhave’s research group, and another team from the École Polytechnique Fédérale de Lausanne (EPFL)—headed by Tobias Kippenberg, professor of physics and head of laboratory photonics and quantum measurement at the Swiss technical university—have replaced the magnetic materials with a piezoelectric thin film for an all-electrically-driven solution. This breakthrough innovation was detailed in a paper, Magnetic-Free Silicon Nitride Integrated Optical Isolator, published on October 21 in Nature Photonics.
Piezoelectric refers to the ability of certain materials to generate an electric charge in response to applied mechanical stress, and vice versa. By driving three piezoelectric micromechanical system (piezoMEMS)-actuated acousto-optic modulators, they mimic the Faraday effect using a technique known as spatiotemporal modulation, eliminating the need for an external bias magnet.
“This design creates an effective rotating acoustic wave, which allows indirect interband transition in only one direction among a pair of strongly coupled optical modes.” Hao Tian explains, who is one of the first authors. Tian adds, “The strong mode coupling creates Rabi mode splitting and a transparency window in the forward direction whereas, in the backward direction, it remains as a single resonance and absorbs the input light. This non-reciprocal transmission property enables us to create electrically driven linear optical isolators.”
“This is the first demonstration of a magnetic-free, electrically driven linear optical isolator on-a-chip, using Complementary Metal-Oxide Semiconductor (CMOS)-compatible fabrication technology and silicon-nitride (Si3N4) photonic integrated circuits (PICs),” says Bhave, who is also associate director of operations at the Birck Nanotechnology Center at Purdue.
The Birck Center includes a 25,000-sq.-ft. ISO (International Organization for Standardization) Class-6 nanofabrication cleanroom, where researchers from six Purdue academic colleges work at the forefront of photonics, nanoelectronics, energy, nanobiological technology, micro-electromechanical systems and nanomanufacturing. The magnetic-free linear optical isolator devices were fabricated at the Birck Center and the EPFL Center of MicroNanoTechnology. The piezoelectric aluminum nitride (AlN) was deposited by Plasma-Therm LLC.
The Purdue and EPFL research is supported by the United States National Science Foundation, the Swiss National Science Foundation, the U.S. Air Force Office of Scientific Research (AFOSR), and the European Union Horizon 2020 program.
“Our technology is wavelength-agnostic, and can be readily adapted to visible and UV light. This is where many atomic clock, quantum sensor and atom-computing platforms are being pursued, and where researchers would like to eliminate the bias magnet in traditional isolators because of the unwanted Zeeman shifts,” Bhave says, and adds, “Our innovation also advances the manufacture of integrated photonics at scale, which can open more opportunities for new applications in blazing fast light-based computing.”