20 minutes with C. Richard Liu

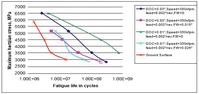
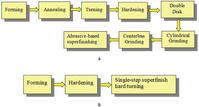
C. Richard Liu - The Quick File
Dr. C. Richard Liu is a professor of industrial engineering (Manufacturing and Materials Group) at Purdue University and an ASME Fellow. He was educated in mechanical and manufacturing Engineering and currently works on research projects on additive manufacturing at nano and conventional scales. His nano-manufacturing research is based on chemical synthesis stimulated by pulsed laser invented by his research group.
Liu is considered a pioneer of several sub-areas of manufacturing research and technology innovation. One sub-area is manufactured surface integrity, which has evolved into a global field of interest. Liu has received several awards, including 2011 SME/NAMRI Wu Research Implementation Award, 2008 ASME Ennor Manufacturing Technology Award, 1985 IR100 Award (as a member of a NIST team) and ASME Blackall Award.
He also has influenced the global manufacturing industry as a pioneer who proposed a global-supply chain structure to Taiwan, which evolved quickly to China and transformed global manufacturing structure. Subsequently, the management of global supply chains has become an added field in IE and business schools. He proposed to Taiwan, RoC, for building the manufacturing industry as a global supplier of key components based on flexible automation technologies in 1980, resulting in a program with about $75 million annual funding for 36 years and continuing.
Liu has published more than 180 peer-reviewed research papers and taught courses including design, manufacturing, mechanics and materials; competitive strategies for products and processes; and structured engineering innovation. He has developed new methods and applied them for more than 30 years for teaching engineering synthesis and design with structured, semi-structured and experience-based approaches.
He has been with Purdue University, starting as an associate professor in 1978, becoming a professor since 1985. He was associate head of graduate program and director of global program from 2007-2011. In his sabbatical leave of three years, he served as vice president, Industrial Technology Research Institute, Taiwan, RoC, from 1997-2000. In his early career, he worked as a research engineer at Whirlpool Corp. where he developed a dominate product. This experience induced his life-long interest in looking for more effective methods of teaching synthesis, design and innovation.
TLT: How are the surface properties of load-carrying components and structures affected by manufacturing processes? What is the disciplinary area studying it?
Liu: New surface formation always will require extra energy. Thus, every manufacturing process creating new surfaces will change the properties of the surface layer material. The engineering discipline for studying this change is called surface integrity. This change can impact significantly the functional performances of the surfaces, such as fatigue. The change is typically characterized by residual stress, micro-hardness, micro-structure, surface finish and surface micro-defect.
Surface residual stress is possibly the most important factor affecting the fatigue life of load-carrying materials and components. When residual stresses are present in a surface, they can positively or negatively impact the performances of the surface under loading. Negative impacts of residual stresses can include significant fatigue life reduction, cracking, warping, dimensional change during machining and grain boundary attack with corrosion.
However, compressive stresses introduced on the surface layer of components can immensely improve fatigue life. Material microstructure (or micro-hardness) also has considerable effect on fatigue life. However, the effect of surface finish produced by modern manufacturing practice is less significant. The surface micro-defects of modern engineered materials are consistently small and is beyond the control of application engineers.
TLT: What is your role in establishing this discipline of surface integrity?
Liu: I have pioneered to establish the scientific foundation in the area of machined surface integrity, along with an innovative application in hard machining. Machining processes are typically used as surface finish or semi-finish processes. As such, they will most significantly impact the surface performances and, thus, dominated the study of surface integrity.
The complex dynamic interactions among heat, deformation and material properties due to chip-removal process result in a new surface created with a residual stress field and possibly alternated microstructures. Typical factors affecting residual stress include the moving stress and temperature fields generated during the pushing of the cutting tools in machining processes. Material properties such as Young’s Modulus, hardness and yield strength in the entire dynamic temperature field will follow the temperature-dependent 3D constitutive stress-strain relationship into plastic region, which has an important role in the final residual stress formation.
My pioneering study of machining-induced surface alteration is the first that identified the root mechanism affecting the integrity of a machined surface. My doctoral research (1), under the late legendary professor Moshe Barash, discovered that the effects of the residual stresses in a machined surface correlated to the length of the shear plane in the chip removal processes with deterministic tools (2). I also proposed a mechanism of residual stress formation in each and all material points below the machined surface, which is due to the sequential elasto-plastic loading and unloading resulting from the moving tool for removing the chip. Thus, the residual stress in a machined surface is dominated by how the chip is formed, with its history of the mechanical and thermal elasto-plastic loading-unloading in the dynamics of chip formation (3, 4).
When machining with a worn tool, the significant effect of the tool wear on surface integrity is due to not only its effect of friction generated by the sliding on the newly created surface but also its effect of the heat on the entire chip formation/removal process (5). This understanding discouraged the research/engineering efforts at that time for correlating the surface integrity with friction due to the fast sliding of the flank face of the tool tip, as reflected by a vast number of publications surveyed by Metcut, Inc. (6).
Though no consideration was found in these papers in linking the state of residual stresses to the dynamics of chip formation, my finding firmly pointed to this new direction of research, which requires not only the inclusion of the entire dynamic chip formation/removal process but also extending the plastic boundary to below the machined surface for studying the surface integrity, as adopted since then. The finding also has led a new generation of metal-cutting research extended to what happens below the machined surface, for which the classic metal-cutting theories (e.g., established by generations led by Gene Merchant and Milton Shaw) ignored.
Thermal energy generated during material removal is detrimental to the surface integrity. A large percentage of machining energy is released as heat when the material is being removed. The greatest temperatures and expansion occur near the surface of the workpiece. Additionally, the overheating and rapid cooling of the surface layers can lead to under-tempered martensite (UTM), over-tempered martensite (OTM) and surface cracks. UTM is produced by overheating the surface layer and is then followed by rapid quenching. UTM is harder and more brittle than the base material; when present, it is typically found on the workpiece’s top surface. OTM also comes from overheating but is followed by slow cooling. It is softer and typically found under the UTM layer. Along with surface cracks, these three conditions have all been shown to significantly reduce fatigue life.
A new methodology was developed to model the thermal effect during machining with a dull tool. By incorporating a microstructure-based experimental method with the thermal model, the heat generated, the heat partition and the shear forces at the tool-chip and tool-work interfaces can be determined. Very precise boundary conditions of interface temperatures that are extremely difficult to measure can be obtained. This method can be used for characterizing, designing and selecting the cutting tools, based on the heat partition among the tool, work and chip during machining, aiming to maximize the heat partitioned into the chip, thus eliminating the thermal induced damage to microstructures (7).
TLT: How have manufacturing processes evolved over the years when dealing with machining hardened steels?
Liu: The processes have started with the need/requirement of precision manufacturing for many finish and super-finish processes, such as precision grinding, honing, super-finish, etc., all using abrasives of random distributions in size and shape, which would not and will not result in consistent favorable residual stresses and microstructures in the machined surface layer, leading to a high probability for fatigue cracks initiated at the weakest material points with locations impossible to predict.
Further, the metal-removal rates are low, geometry generation is not flexible, processing time and processing cost are high. Though the situation has improved with ongoing innovative super-hard abrasives that may reduce harmful tensile residual stresses and decrease brittle microstructures due to more favorable frictional and heat transfer behavior in abrasive materials, the overall situation still offers further innovative opportunities.
My research group, supported by the National Science Foundation and industry, invented a processing concept of using deterministic machining processes alone to complete all the machining requirement, roughing and finishing, thus eliminating the needs of using abrasive machining for finish and super-finish operations. We further successfully pioneered methods of modeling 3D hard machining for predicting residual stresses and microstructural alterations (8, 9). For my contribution to the understanding of surface integrity of a machined surface and the pioneering research on hard machining, I was awarded in 2008 the prestigious ASME Ennor Manufacturing Technology Award.
TLT: For your innovative research on finish/super-finish hard turning, you also were awarded the prestigious S. Wu Research Implementation Award in 2011 by North American Manufacturing Research Institute/Society of Manufacturing Engineers. Can you explain the potential impact of this awarded work?
Liu: This award stresses more of the impact of research implementation. The award citation may summarize the potential impact:
“The award recipient, Professor C. Richard Liu, is recognized for having created single-step finish/superfinish hard machining for performing roughing, finish and superfinish processes within a single setup, a new market and a science base for engineering a new generation of processes, machine tools, cutting tools and manufacturing systems. This research has been successfully applied in manufacturing load-carrying components and has led to significant benefits of reduced cost and improved efficiency, flexibility, controllability and quality for manufacturing load-carrying components, resulting in higher component fatigue life, system reliability and safety and environmental sustainability.”
TLT: How does the new process improve the fatigue life of the machined components made of hardened steels?
Liu: It was shown by our research, for the first time, that the residual stresses in a machined subsurface will become more compressive as the hardness of the workpiece is increased (10). This was considered an incentive for the subsequent research effort for eliminating the re-quenched brittle layer and re-annealed softer layer, both detrimental to fatigue life, possibly associated with all finish machining of hardened steels, including hard turning. With NSF funding, we have shown success.
After establishing the ability to eliminating microstructure alteration and, in the meantime, deep compressive residual stresses producible, hard machining thus can be used as a finish process. Subsequently, the concept of producing pre-stressed components to offset the effect of external loading and a method of manufacturing an optimally pre-stressed surface by machining were developed (11). It was shown experimentally that this new concept could be very effective as the fatigue life of a machined surface can vary as much as 35 times by using different machining conditions as shown in Figure 1.
TLT: What are the economic and social impacts of the single-step hard machining?
Liu: On economic impact: load-carrying components are of critical importance for advanced products including jet engines, aircrafts, ships, automobiles, nuclear power plants, etc. The economic impact on the industries making/using these components is enormous, considering the significant annual sales of the products.
It also was shown by our group that residual stress variations due to grinding were at much higher scales than that due to machining with deterministic tools in a series of publications for facing and grinding hardened steels (12) and drilling, facing and grinding Ti Alloys (13). In the meantime, we proved that the fatigue life variation is almost entirely due to the residual stress variations for a given component without structural alteration (14). These understandings pointed to worthy research efforts for enabling deterministic machining as finish/superfinish processes replacing grinding, for which we have succeeded.
A preliminary study showed that finish hard turning was able to reduce the cost by 30%-40% while reducing the in-factory time by half for machining a hardened component in small lot size production. The overall simplification in general is shown in Figure 2.
There are additional benefits created by improved fatigue life with reduced variance. These benefits include increased safety and reduced inspection and repair. However, there is no estimation for their economic impact at this time. Since 1996 research and applications of hard turning as a finish process have increased significantly in academia and industry in the U.S. and Europe. A notable development that occurred later than 1996 was a project funded by the NIST ATP program, under the leadership of Delphi Automotive, partnered with Kennametal, Hardinge, Torrington and Georgia Tech. This project was structured by Delphi, after funding me a small project, consistent with my suggested directions and received additional consultation from me.
It is known that finish hard turning has been applied by several American multinationals, benefiting the production of bearings for helicopters, automobiles, bullet trains and other products. This production concept also has been presented widely by me to many world-class companies with substantial interaction or research funding, including Timken, Caterpillar, Delphi Automotive, GM, Ford, Brenco and Spirit Aerosystems. The applications are still spreading.
On load-carrying capacity and fatigue life: our research has shown that resultant fatigue lives of rolling contact of the manufactured surfaces can vary by 50 times between the best finish hard turned surface and a typically ground surface. Equally significant, the fatigue life can vary as much as 35 times even among the finish hard turned surfaces using different turning conditions (12). This result has clearly shown that very significant improvements are possible by carrying out the proposed process optimization disclosed in a 1999 patent and enabled by subsequent research.
On safety: the new process proposed by me is shown to significantly improve fatigue life not only by increasing the mean value but also by the reduction of variance (12, 15). This result will facilitate better scheduling for inspection and repair with enhanced safety at much reduced cost.
TLT: The citation of the SME/NAMRIC Wu Research Implementation Award includes the following phrase: “having created a new market and a science base for engineering a new generation of processes, machine tools, cutting tools and manufacturing systems.” Can you offer more explanation to our readers?
Liu: The potential economic and social impact of the single-step hard machining technology provides incentives for pursuing the following technical opportunities to gain the benefits of a new market segment: (1.) the development of new processing concept and applications taking advantage of hard machining processes, (2.) the development of cutting tools that have proper frictional and heat transfer properties for hard machining, (3.) the development of machine tools with proper precision/accuracy to fully gain the benefits of finish hard machining and the development of new manufacturing systems eliminating the inflexible, slow, costly finish processes to gain the full benefit of CAM/CAM integration and the benefit of flexibility, remote controllability over internet and reduced cost. We are happy to report that these developments have indeed happened.
REFERENCES
1. Liu, C. (1973), “An Investigation of the Surface Condition in a Steel Component Machined by Chip Removal Process,” PhD thesis, Purdue University.
2. Liu, C.R. and Barash, M.M. (November 1976), “The Mechanical State of the Sublayer of the Surface Generated by Chip-Removal Process, I: Cutting with a Sharp Tool,” ASME Transactions, Journal of Engineering for Industry, 98, Series B, 4, pp. 1192-1201.
3. Liu, C.R. and Lin, Z.C. (1985), “Effects of Shear Plane Boundary Condition on Stress Loading in Orthogonal Machining,” International Journal of Mechanical Sciences, 27 (5), pp. 281-290.
4. Lin, Z.C., Lin, Y.Y. and Liu, C.R. (1991), “Effect of Thermal Load and Mechanical Load on the Residual Stress of a Machined Workpiece,” International Journal of Mechanical Sciences, 33 (4), pp. 263-278.
5. Liu, C.R. and Barash, M.M. (November 1976), “The Mechanical State of the Sublayer of the Surface Generated by Chip-Removal Process, II: Cutting with a Tool with Flank Wear,” ASME Transactions, Journal of Engineering for Industry, 98, Series B, 4, pp. 1202-1208.
6. Koster, W.P., et al. (April 1973), “Manufacturing Methods for Surface Integrity of Machined Structural Components,” Technical Report AFML-TR-71-258, Metcut Research Associates, Inc.
7. Wang, J.Y., Liu, C.R. and Wang, K.K. (1999), “The Effect of Tool Flank Wear on the Heat Transfer, Thermal Damage and Cutting Mechanics in Finish Hard Turning,” CIRP Annals, 48 (1), pp. 53-58.
8. Guo, Y.B. and Liu, C.R. (May 2002), “3D FEA Modeling of Hard Turning,” Trans. ASME, Journal of Manufacturing Science and Engineering, 124 (89), pp.189-199.
9. Shi, J. and Liu, C.R. (2005), “On Predicting Material Softening Effect in Hard Turning – Part I Construction of Material Softening Model,” Journal of Manufacturing Science and Engineering, 127 (3).
10. Matsumoto Y., Barash, M.M. and Liu, C.R. (August 1986), “Effect of Hardness on the Surface Integrity of AISI 4340 Steel,” ASME Transactions, Journal of Engineering for Industry, 108, pp. 169-175.
11. Liu, C.R. and Mittal, S. (1995), “Single Step Superfinishing Using Hard Machining Resulting in Superior Surface Integrity,” Journal of Manufacturing Systems, 14(2), pp. 129-133.
12. Agha, S.R. and Liu, C.R. (2000), “Experimental Study on the Performance of Superfinish Hard Turned Surfaces in Rolling Contact,” Wear, 244, pp. 52-59.
13. Yang, X., Liu, C.R. and Grandt, A.F. (2002), “An Experimental Study on Fatigue Life Variance, Residual Stress Variance, and Their Correlation of Face-Turned and Ground Ti 6Al- 4V Samples,” Trans. ASME Journal of Manufacturing Science and Engineering, 124 (4), pp. 809-819.
14. Liu, C.R. and Yang, X. (2001), “The Scatter of Residual Stresses due to Machining and Grinding,” Journal of Machining Science and Technology, 5 (1), pp. 1-22.
15. Choi, Y. and Liu, C.R. (2006), “Rolling Contact Fatigue Life of Finish Hard Machined Surfaces: Part 1. Model Development,” Wear, 261, pp. 485-491, ScienceDirect Top 25 Hottest Articles.
Source: Rachel Fowler, Managing Editor, Tribology & Lubrication Technology journal, Society of Tribologists and Lubrication Engineers (STLE), "20 Minutes With C. Richard Liu" (July 2019).