|
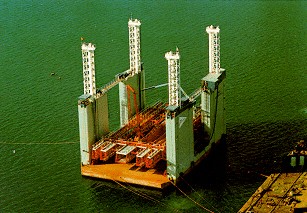
Fig. 1: Floating Dock |
Objectives
The objective of this paper is to provide a summary of the
prefabricating operations for building floating caissons used in the construction of docks
and harbors. A description of the technology, the processes involved and a simulation
model are presented
Floating
Caissons
In certain cities and even countries the demand for land and
space is rapidly exceeding the supply. Maritime centers do not escape from this reality,
the continuous expansion of commercial maritime traffic and activities in seaports due to
increases in international trading has generated an increased demand for an effective use
of ports and harbors. Construction activities have been oriented to the expansion of
existing facilities
Port and harbor facilities form the infrastructure that
makes marine traffic possible, facilitating the construction of vessels, its protection
against wave action and, its loading and unloading activities. In other words, they play
an important role in facilitating international commerce
As mentioned above, a solution to this supply problem has
been the expansion of ports. One of the methods that allows a fast paced construction of
docks is based on the use of floating caissons. The floating caissons are prefabricated
concrete box-like elements with cylinder cavities or cells that are built with the help of
a special equipment named "Floating Docks." The floating caissons dimensions are
customized to each project requirements within certain limits. The floating dock
fabrication equipment studied in this report is a proprietary technology owned by DRAGADOS
(Fig. 1).
The different marine works and harbor constructions in
which these caissons can be used include:
- Ports
- Breakwaters
- Wharves
- Berthing Facilities and Docks
- Dry Docks and Slipways
- Fishing Ports and Marinas
Prefabricating Operation
The prefabricating operation of the caissons starts with the
concrete preparation. Two sets of equipment are used simultaneously covering each half of
the operation. The operation sequence is as follows: A mixer prepares the concrete batch
that is then poured into a hopper that feeds a concrete pump. The concrete is then pumped
to a delivery pipe that is installed above the working deck (Fig. 2).
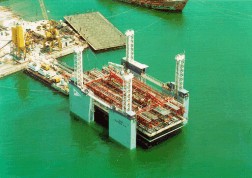
Fig. 2: Floating Caissons Fabrication
Each caisson is built in an ascending
sequence starting with the slab. The slab reinforcement cage is assembled in an auxiliary
floating platform, then the cage is moved to the floating dock. A sliding form is placed
and the slab is poured as a monolithic element
After the slab is ready, the construction of the upper part of the
caissons begins, ascending in increments of one meter using the sliding form Each of these
increments includes: placing the reinforcement, sliding the forms, and pouring and
vibrating the concrete. This sequence is repeated until the total height of the caisson is
reached (Fig. 3).
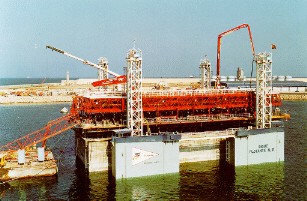
Fig. 3: Floating Caissons Fabrication
Once the caisson fabrication is completed, a
special set of supporting and locking bars are removed to allow the release of the caisson
from the floating dock. The caisson floats by itself and is guided with the help of cables
from the shore and tow-boats, to its final location (Fig. 4). When the caisson reaches the
final position the cylinder cavities begin to be filled with granular material. This
operation is performed by auxiliary floating platforms that carry both the material and a
special crane to transfer the material. Tractors, dozers, loaders and trucks help finish
the filling operation on top of the caisson In the floating dock, operations begin for the
fabrication of the next caisson (Fig. 5).
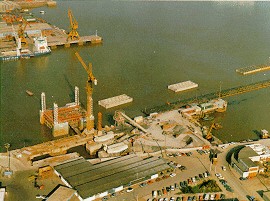
Fig. 4: Floating Caissons Fabrication
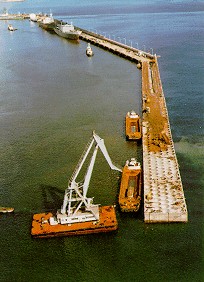
Fig. 5: Floating Caissons Fabrication
Using Floating Caissons for the Expansion of the Port of Valencia
This report is based on the caisson fabrication operations
performed by DRAGADOS for the expansion of the Port of Valencia in Spain. Valencia is
located 352 km southwest of Madrid in the Azahar Coast of the Mediterranean Sea (Fig. 6).
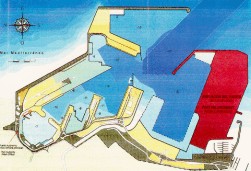
Fig. 6: Port of Valencia Expansion
The project involved the construction of
3,805 m of breakwaters and 996 m of wharves. The new area created between these two
structures was of 120 Ha. (297 Acres). Almost half of Purdue's total area of 650 acres.
This new area required more than 17,000,000 m3 of dirt to be filled.
The 996 m of wharves were constructed using 26 floating caissons
Each floating caisson had the following dimensions: 42 25 m (138.61 ft) long, 15.65 m
(51.35 ft) width, 16.5 m (54.13 ft) height, its concrete volume was of 2,857m3 (3,737 cy),
required 116, 27 metric tons of rebar and had an approximate weight of 10,560 metric tons.
The caisson consisted of a concrete solid slab of 0.6m (1.97 ft) height, followed by a
concrete parallel piped with 62 cylinder cavities, 3 8m (12.47ft) diameter, to reduce its
weight (Fig. 7). DRAGADOS has fabricated a
total of almost 1,000 of these caissons during the last 50 years. Their experience has
allowed them to successfully develop and continuously improve this technology.
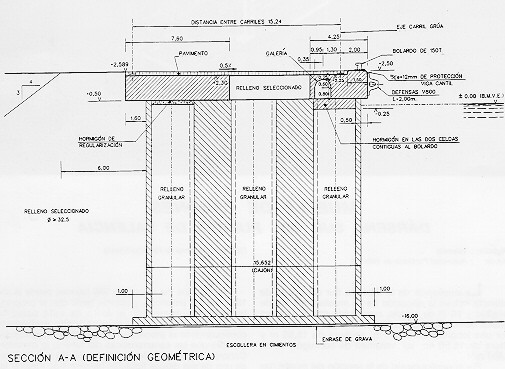
Fig. 7: Floating Caissons - Longitudinal Section
Analysis of the Main
Processes
By observing the prefabricating operation above
described and considering the durations and resources involved, four processes or cycles
were determined to be relevant for the operation's production, these were:
- Concrete Mixing and Pumping
- Slab Pouring
- Upper Caisson Pouring
- Retrieval of Support Bars
Besides the traditional materials required in concrete
construction, the resources involved in the fabrication operation include:
- The Floating Dock that holds two Concrete Delivery Pipes and a Crane
- Two Auxiliary Floating Platforms
- Batch Plant
- Two Concrete Pumps
- Gantry Crane
- Loaders
- Air Compressors
- Pneumatic Pumps
- 34 Workmen per 3 Shifts
Of the four main processes being considered the Upper Caisson
Pouring was chosen for study It was the most relevant processes, considering the amount of
work involved It represented 85% of the concrete being poured and an estimated 74% of the
fabricating operation's time.
The analysis of the process cycle was conducted when the third
caisson was under fabrication. The recorded total fabrication cycle for the two first
caissons averaged 153 hr. 39 hr above the established 114 hr when construction was
planned. Considering that each day had a production cost of PTA 2.5M (US$18,700)
improvements needed to be made.
A flow unit of 1 cubic meter of concrete was established for
modeling purposes. Since this process cycle depended on the concrete mixing and pumping,
cycle capacities were checked to make sure that they did not represent a bottleneck The
capacities obtained were:
Concrete Mixing Process: |
Plant 65 m3/hr |
Pumping Process: |
Pump 1 53 m3/hr |
Pump 2 31 m3/hr |
The Upper Caisson Pouring process registered
a productivity of 35.7 m3/hr, very much below the 65 m3/hr that is possible to produce and
pump.
Development of the CYCLONE
Model
Considerations:
- The upper caisson is poured in layers of 25 cm (38 m3). For this
volume each pump must discharge 19 m3 of concrete, equivalent to 12.7 batches.
- The concrete delivery pipes, have to interrupt their pouring process
12 times each, due to the steel beams of the working deck that restrict their free
movement. This means that each delivery pipe will discharge approximately 1.1 batches
before changing its position.
- The concrete plant prepares batches that are directed to each pump
as needed.
The model developed for this operation is shown in Fig. 9 with the
PROSIDYC simulation reports in the following pages. The results of these runs will be the
basis for comparisons between proposed alternatives.
Proposed Alternatives
- Alternative 1: Double the capacity of the pump
hoppers allowing them to receive each, two batches from the concrete plant. The results
and reports from this alternative can be seen in Fig. 10.
- Alternative 2: Consists of alternative 1 plus the
reduction in the number of Delivery Pipe positioning from 12 to 8. This could be done by
modifying the shape of the working deck structure beams. Instead of using a beam with a
rectangular section, a V section could be used in order to have a large area to pour
concrete without repositioning the delivery pipe. The results and reports from this
alternative can be seen in Fig. 11.
Results
The productivity obtained in each of the models are the following,
considering 100% and 90% time efficiency:
Time Efficiency: |
100% |
90% |
Original Situation |
34 m3/h |
31 m3/h |
Alternative 1 |
42 m3/h |
37 m3/h |
Alternative 2 |
47 m3/h |
42 m3/h |
Conclusions
and Recommendations
- The productivity of the process was increased in 24% by doubling the
capacity of the pump hoppers.
- Alternative 2 considered the double capacity of alternative 1 and
also a modification in the working deck structure, by changing the section of its beams,
from a rectangular shape to a V shape. Allowing a larger pouring area which reduced the
number of positions for the delivery pipes. This modifications showed an increase in the
productivity of 38% when compared to the original process or 14% over Alternative 1.
- A cost analysis of the structural modification to the working deck
is recommended, comparing the cost benefits that could be received by an improvement of
only 14% of the productivity, to the cost of such structural modifications.
- In the models used in this analysis, two pumps with different
capacities were considered. This created an uneven concrete assignment from the plant
which translated in an uneven concrete pouring task for each area. The use of two equal
capacity pumps should be studied.
- The capacity of both pumps is superior to the capacity of the plant,
thus a further study is recommended to optimize such relation.
|