
Background
Education
- Dalian Institute of Chemical Physics, Chinese Academy of Sciences, Ph.D. Physical Chemistry (2010)
- Hunan University, M.S. Material Physics and Chemistry (2006)
- Hunan University, B.S. Applied Physics (2003)
Experience
- Purdue University, Research Scientist (June 2019 - present)
- Purdue University, Postdoctoral Reseacher (Jan. 2013 - May 2019)
- Argonne National Laboratory, Postdoctoral Reseacher (Jul. 2012 - Jan. 2013)
- Technical University of Denmark, Postdoctoral Reseacher (Apr. 2010 - Jun. 2012)
Teaching
- Purdue University, Spring 2024 CHE 43500 Chemical Engineering Laboratory
- Purdue University, Fall 2023 CHE 37700 Momentum Transfer (Fluid Mechanics)
- Purdue University, Spring 2022 CHE 20500 Chemical Engineering Calculations
- Purdue University, Spring 2022 CHE 21100 Introductory Chemical Engineering Thermodynamics
Research Description
Research Philosophy 1:
Seek not to imitate the masters; Seek what they sought
Research Philosophy 2:
Everything should be made as simple as possible, but no simpler
Vision:
Precise Computational Design of Advanced Electrocatalysts for Hydrogen Energy and Carbon Negative Technologies
Mission:
To Drive Self-Consistent Modeling and Fundamental Understanding of Real-World Electrochemical Interfaces
Definition:
Self-consistent modeling corresponds to the Operando experiment with the following characteristics.
- [I] Identify active phases and atomic structures of the catalysts that existed under reaction conditions
- [II] Evaluate steady-state surface configurations of active phases under reaction conditions
- [III] Consider reaction centers and catalytic mechanisms on steady-state surfaces of active phases
- [IV] Cross-check each step with measurement. If the predictions are consistent with the measurements, then self-consistently identify the active phases, steady-state surface structure, reaction center and catalytic mechanism under experimental conditions. Otherwise, consider additional possibilities based on the measurements.
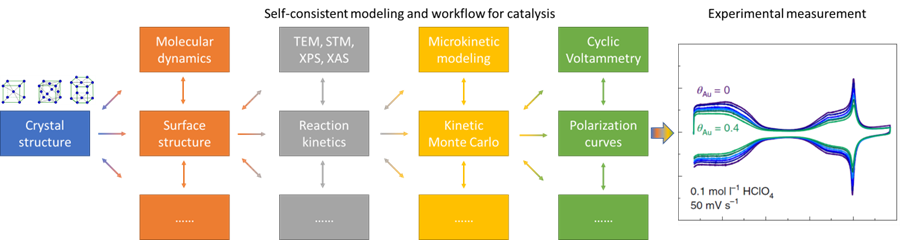
Definition:
Real-World Electrochemical Interfaces include complex metal-metal interfaces, metal-oxide interfaces, oxide-oxide interfaces and solid-liquid interfaces. Specifically, I will focus on the following types of interfaces.
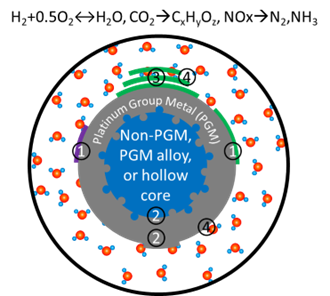
- 1. Metal-oxides interfaces: intelligent catalysts for electrochemical energy conversion
- 2. Metal-Metal interfaces: structural evolution of epitaxial film electrodes and core@shell nanocatalysts
- 3. Oxide-oxide interfaces: active phases and reaction centers of transition metal oxyhydroxides for the oxygen evolution
- 4. Solid-liquid interfaces: rigorous modeling of electrified electrolyte/electrode interfaces
1. Metal-oxides interfaces: intelligent catalysts for electrochemical energy conversion
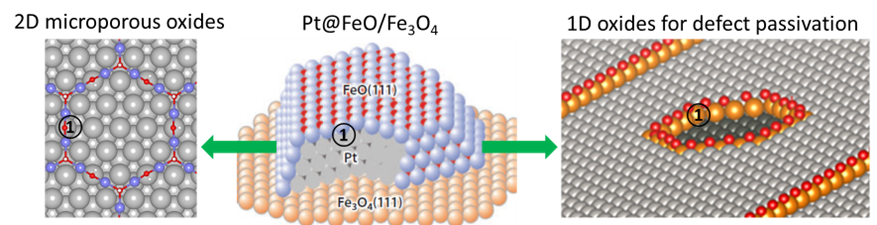
Improving catalytic activity, stability and selectivity are the major goals for the hydrogen energy and carbon negative efforts toward net-zero emission. The strong metal-support interaction (SMSI) phenomenon is a classic concept in heterogeneous catalysis, which is characterized by the encapsulation of metal nanoparticles by ultrathin oxide films. While full encapsulation would deadly suppress catalytic activity, it could be taken advantage of to develop intelligent catalysts with unique selectivity, stability and catalytic activity for electrochemical energy conversion (including oxygen cycle, carbon cycle and nitrogen cycle). Those goals can be achieved through a fundamental understanding of the preferential formation of the following type of interfaces: [1] 2D dense oxide/metal interfaces, [2] 2D microporous oxide/metal interfaces, [3] 1D oxide/metal interfaces. While forming 2D dense oxides will block active sites, preferential blocking may tune the selectivity and eliminate byproducts. On the other hand, forming 2D microporous oxides/metal interfaces may lead to bi-functional catalysts with improved activity and stability. Also, defect passivation through the preferential formation of 1D oxides is crucial for the long-term performance of electrocatalysts used under harsh electrochemical environments, e.g., in fuel cells.
2. Metal-metal interfaces: structural evolution of epitaxial film electrodes and core@shell nanocatalysts
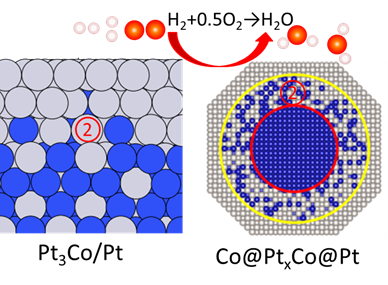
Activity and durability (cyclability) have been major challenges in electrochemical devices toward achieve net-zero emission. Recent studies have shown that catalytic activity of epitaxial Pt films (shells) strongly depends on its thickness for a variety of electrocatalytic reactions, e.g., hydrogen oxidation and oxygen reduction in fuel cells. The goal of this project is to study interface structure and strain evolution of epitaxial noble metal electrocatalysts with various thickness and, as an extension, real-world core@shell nanocatalysts. As the thickness of the noble metal overlayers is crucial to both the activity and the stability (i.e., protecting dissolution) of the catalyst, the present study will lead to principles for precise design of electrocatalysts with optimal activity and stability. The fundamental understanding also can be used to study metal-air batteries and establish the principles of high cyclability.
3. Oxide-oxide interfaces: active phases and reaction centers of transition metal oxyhydroxides for the oxygen evolution
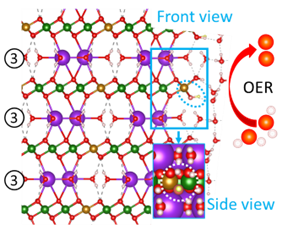
Oxygen evolution reaction (OER) is the bottleneck of water electrolysis for hydrogen generation and metal-air batteries. Because it happens under extremely oxidative aqueous conditions, in-situ crystal structures of the OER active phase are largely unknown, which has significantly hindered the establishment of structure-property relationships and, consequently, the design of new catalysts. The goal of this project is to self-consistently identify the catalytically active phases, reaction centers and OER mechanisms of transition metal oxyhydroxide electrocatalysts. An effective error alignment and error cancellation strategy will be employed to accurately describe strongly-correlated transition metal oxides. A simulated annealing method will be developed and used to approach global minima and identify active phases. The identified atomic-scale structures will be used to study the steady-state surface phases, reaction centers, and catalytic mechanisms. To verify the fundamental understanding, spectroscopy (e.g., XAS) simulation will be performed to link modeling with measurements from collaborators. By combining a large phase space with machine learning algorithms, multifunctional reaction centers capable of breaking the scaling rules that determine volcano plots with designed through tuning the composition, the geometric and electronic structures (polaron) of the active phases.
4. Solid-liquid interfaces: rigorous modeling of electrified electrolyte/electrode interfaces
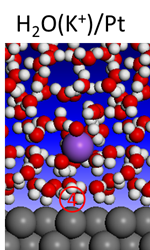
For aqueous electrocatalysis, in comparison with heterogeneous catalysis at gas/solid interfaces, the effect of solute, solvent, pH, and electrode potential at electrified interfaces make modeling and consequent design more complicated. The goal of this project is to combine ab initio molecular dynamics simulation with our recently developed electronic-structure-based energy level alignment and electrode potential control techniques to advance new insights for the rigorous modeling of electrified water/solid interfaces. This understanding and methodologies developed will make it possible to include all the double-layer features (e.g., hydrogen bonds, cation effect and electrode potential) in the ab initio simulation. The development will eventually be integrated into the above efforts to produce detailed treatments of complex reactions. The ultimate goal is to identify a series of multifunctional catalysts with enhanced activity, selectivity, cost efficiencies, and resistance to degradation for energy-critical electrochemistry and heterogeneous catalysis.
Perspective
The coming three decades will present many exciting opportunities for the study of hydrogen energy and carbon negative technologies. Those efforts will be accelerated through the precise computational design of advanced electrocatalysts, which is further driven by self-consistent modeling and fundamental understanding of real-world electrochemical interfaces.
Awards
- Singapore NRF Fellowship Finalist (2019)
- ECS - Toyota Young Investigator Fellowship [Press Release]
- Psi-K Conference Travel Award (2010)
Recent Publications
- G. Liu*, A. J. Shih*, H. Deng, K. Ojha, X. Chen, M. Luo, I. T. McCrum, M. T. M. Koper, J. Greeley†, Z. Zeng†, Site-specific reactivity of stepped Pt surfaces driven by stress release. Nature 626, 1005-1010 (2024).
- Kaustubh Sawant, Zhenhua Zeng†,Jeffrey Greeley, Origin of Stability and Activity Enhancements in Pt-based Oxygen Reduction Reaction Catalysts via Defect-Mediated Dopant Adsorption, Angew Chem Int Ed e202312747, 2023.
- Qianbao Wu, Junwu Liang, Mengjun Xiao, Jing Zhu, Zhenhua Zeng†,Chunhua Cui†, et al, Non-covalent ligand-oxide interaction promotes oxygen evolution, Nature Communications 2023, 14, 997.
- Hee-Joon Chun, Zhenhua Zeng†, Jeff Greeley†, DFT Insights into NO Electrochemical Reduction: A Case Study of Pt(211) and Cu(211) Surfaces, ACS Catal. 2022, 12, 1394
- Zheng Li, Yang Xiao, Zhenhua Zeng, Jeff Miller, Jeff Greeley, Yue Wu, et al, Direct methane activation by atomically thin platinum nanolayers on two-dimensional metal carbides, Nature Catalysis 2021, 4, 882
- Junxian Gao, Kaustubh Sawant, Jeff Miller, Zhenhua Zeng†, Dmitry Zemlyanov†, Jeff Greeley†, Structural and Chemical Transformations of Zinc Oxide Ultrathin Films on Pd(111) Surfaces. ACS Applied Materials & Interfaces 2021, 13, 35113
- Fabio Dionigi*†, Jing Zhu*, Zhenhua Zeng†, Thomas Merzdorf, Hannes Sarodnik, Manuel Gliech, Lujin Pan, Wei-Xue Li, Jeffrey Greeley, Peter Strasser, Intrinsic Electrocatalytic Activity for Oxygen Evolution of Crystalline 3d-Transition Metal Layered Double Hydroxides Angew. Chem. Int. Ed. 60, 14446-14457 (2021).
- Fabio Dionigi*†, Zhenhua Zeng*†, Ilya Sinev, Thomas Merzdorf, Siddharth Deshpande, Miguel Bernal Lopez, Sebastian Kunze, Ioannis Zegkinoglou, Hannes Sarodnik, Dingxin Fan, Arno Bergmann, Jakub Drnec, Jorge Ferreira de Araujo, Manuel Gliech, Detre Teschner, Jing Zhu, Wei-Xue Li, Jeffrey Greely, Beatriz Roldan Cuenya† and Peter Strasser†, In-situ Crystal Structure and Reaction Mechanism for NiFe and CoFe Layered Double Hydroxides in the Oxygen Evolution Reaction Catalysis , Nature Communications 11, 2522 (2020).
- Lei Wang*, Zhenhua Zeng*†, Wenpei Gao, Tristan Maxson, David Raciti, Michael Giroux, Xiaoqing Pan, Chao Wang, Jeffrey Greeley, Tunable intrinsic strain in two-dimensional transition metal electrocatalysts, Science, 2019, 363, 870
- Z. H. Zeng, K.-C. Chang, J. Kubal, N. Markovic and J. Greeley, Stabilization of Ultrathin (Hydroxy)oxide Films on Transition Metal Substrates for Electrochemical Energy Conversion, Nature Energy, 2017, 2, 17070
† Corresponding authors