RESEARCH
Actuators
Magnetic microactuator enabled self-clearing catheters
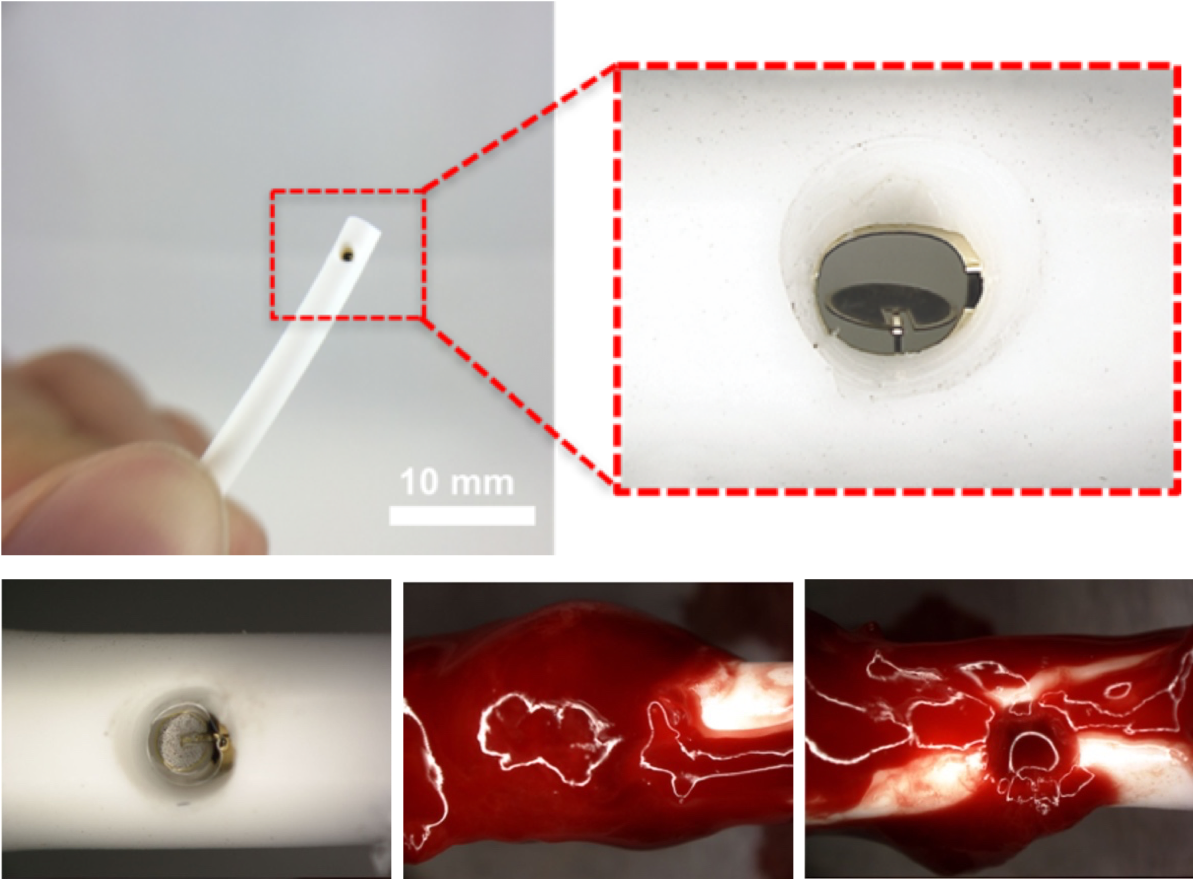
Automatic antidote delivery system for treatment of opioid overdose
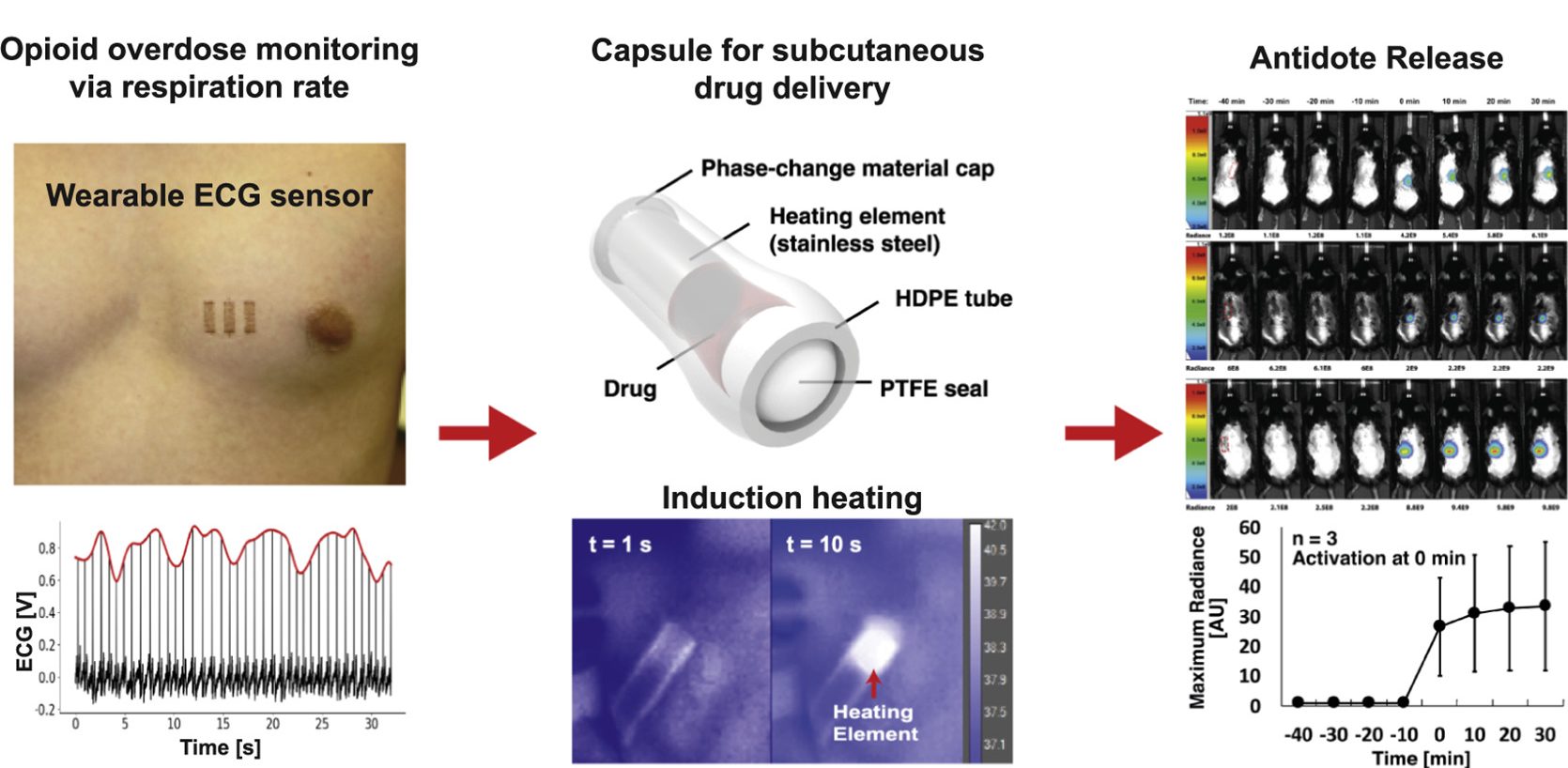
Magnetic actuator-enabled 3D cell culture system for cancer research
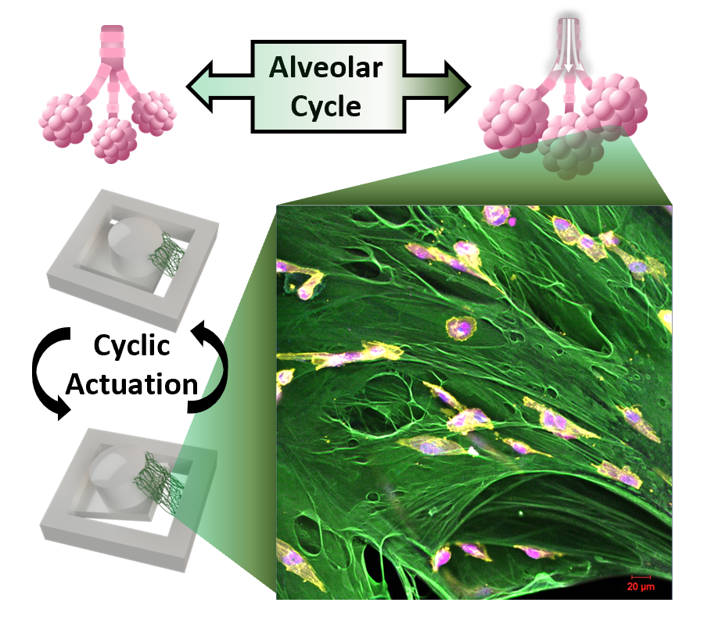
Sensors
Printable implantable glutamate biosensor using conductive Pt nanoparticle composite ink
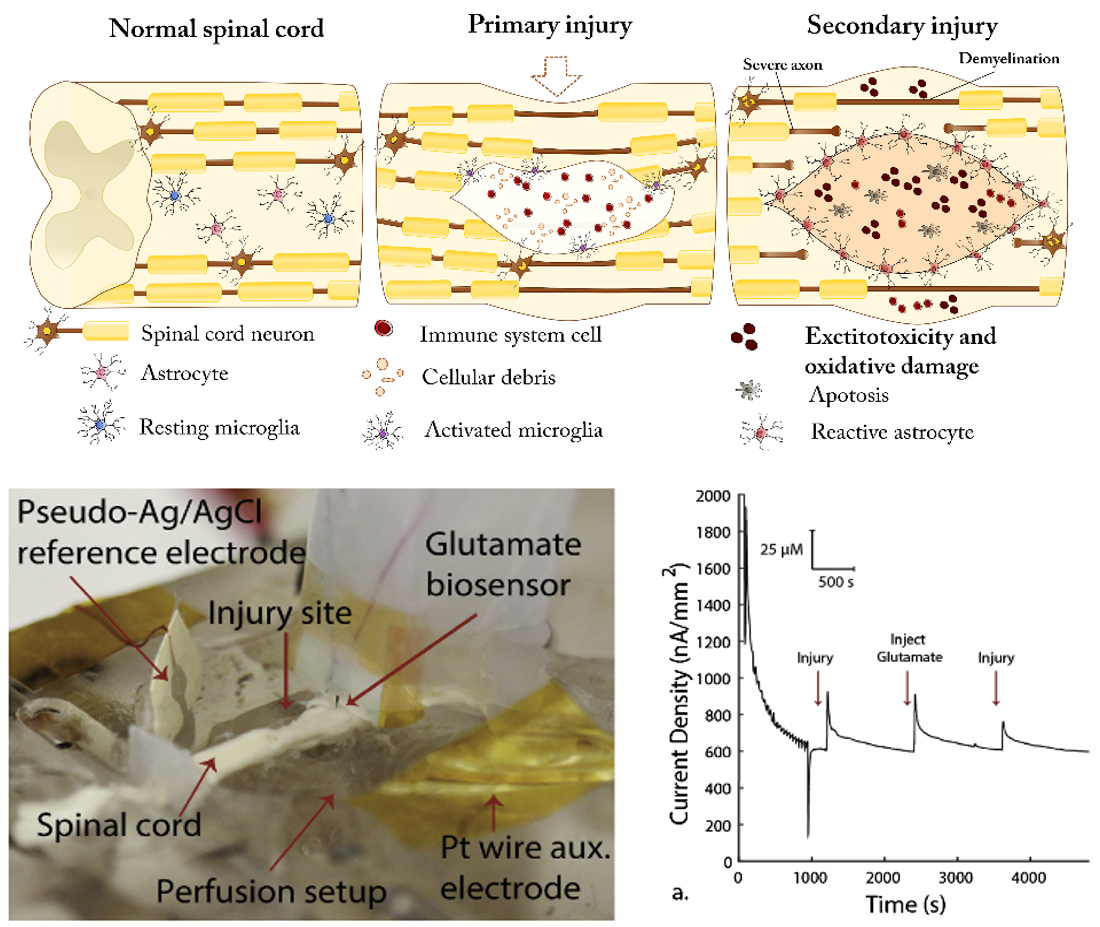
Activated carbon-Pt based printable glutamate biosensor
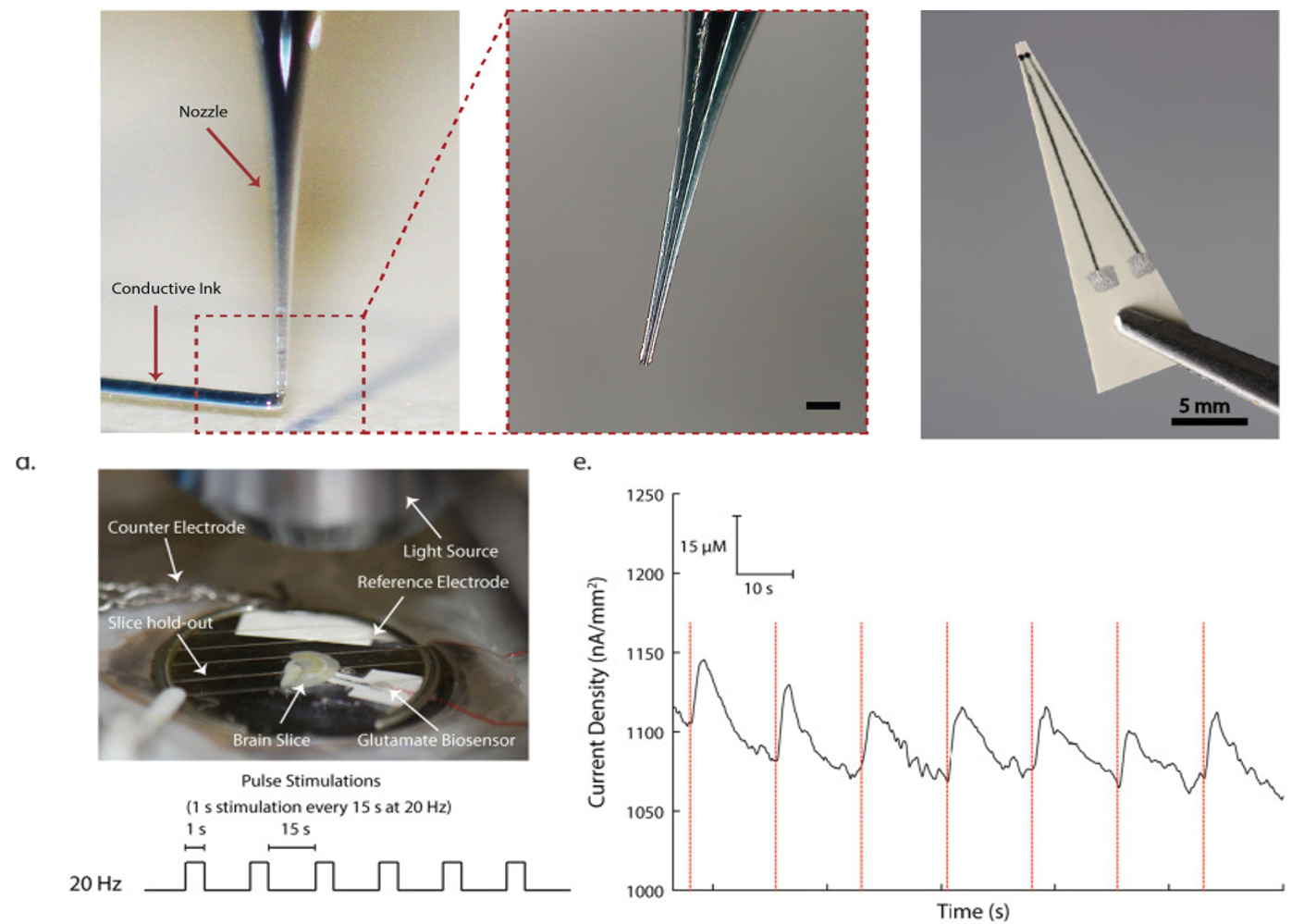
Multi-analyate biosensor array
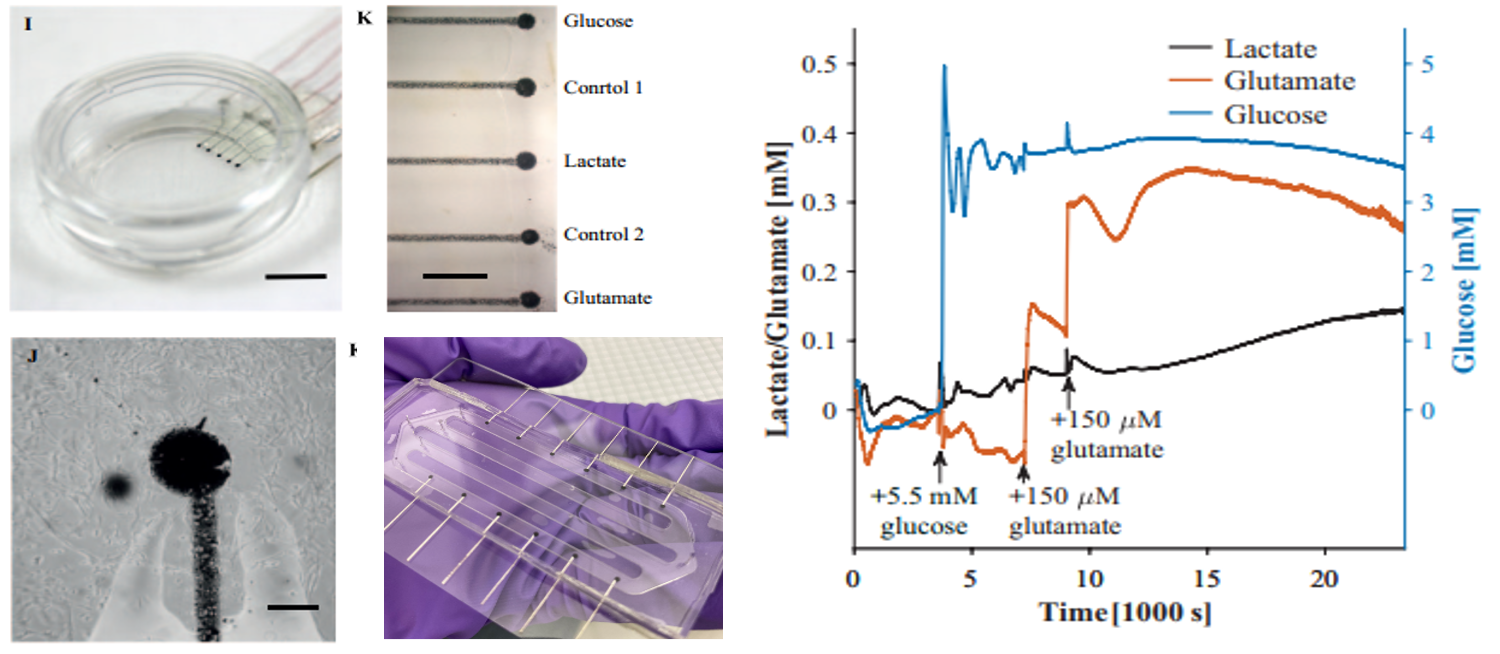
CNT-PtNP based non-enzymatic glucose biosensor and the non-linear modeling
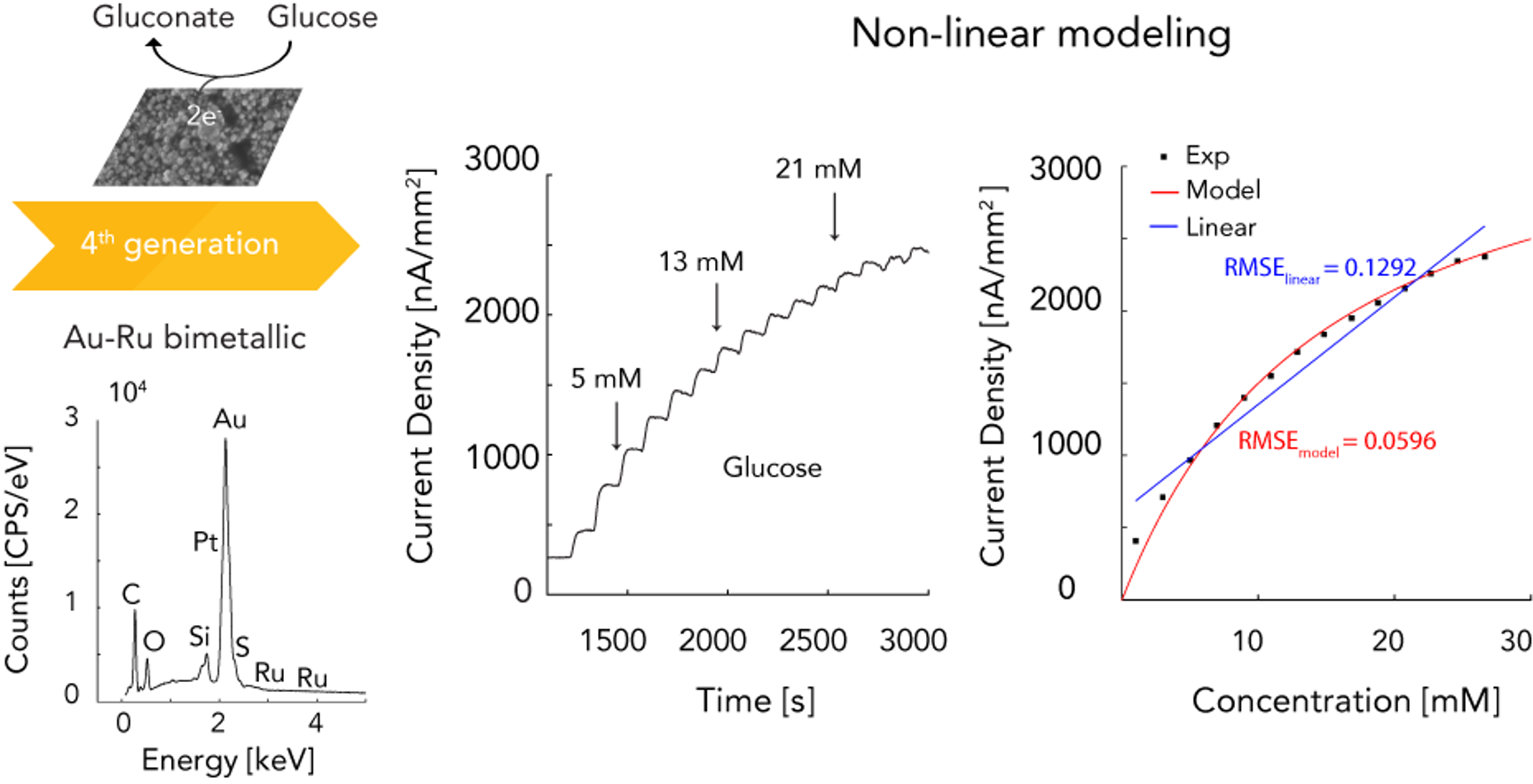
Perovskite-based implantable glutamate biosensor for in vivo cortical monitoring
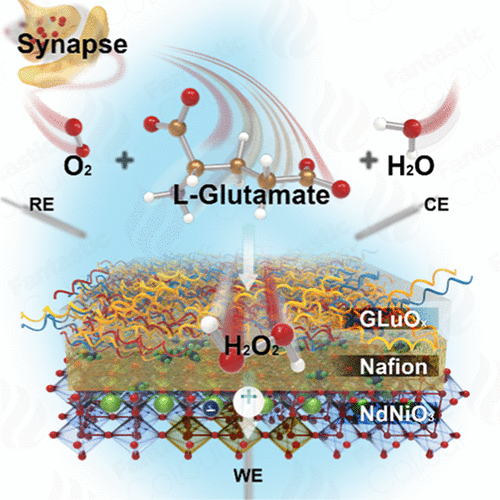
Anti-biofouling strategies
Simple fabrication technique to create a large-scale, anti-inflammatory biomimetic nanotexture
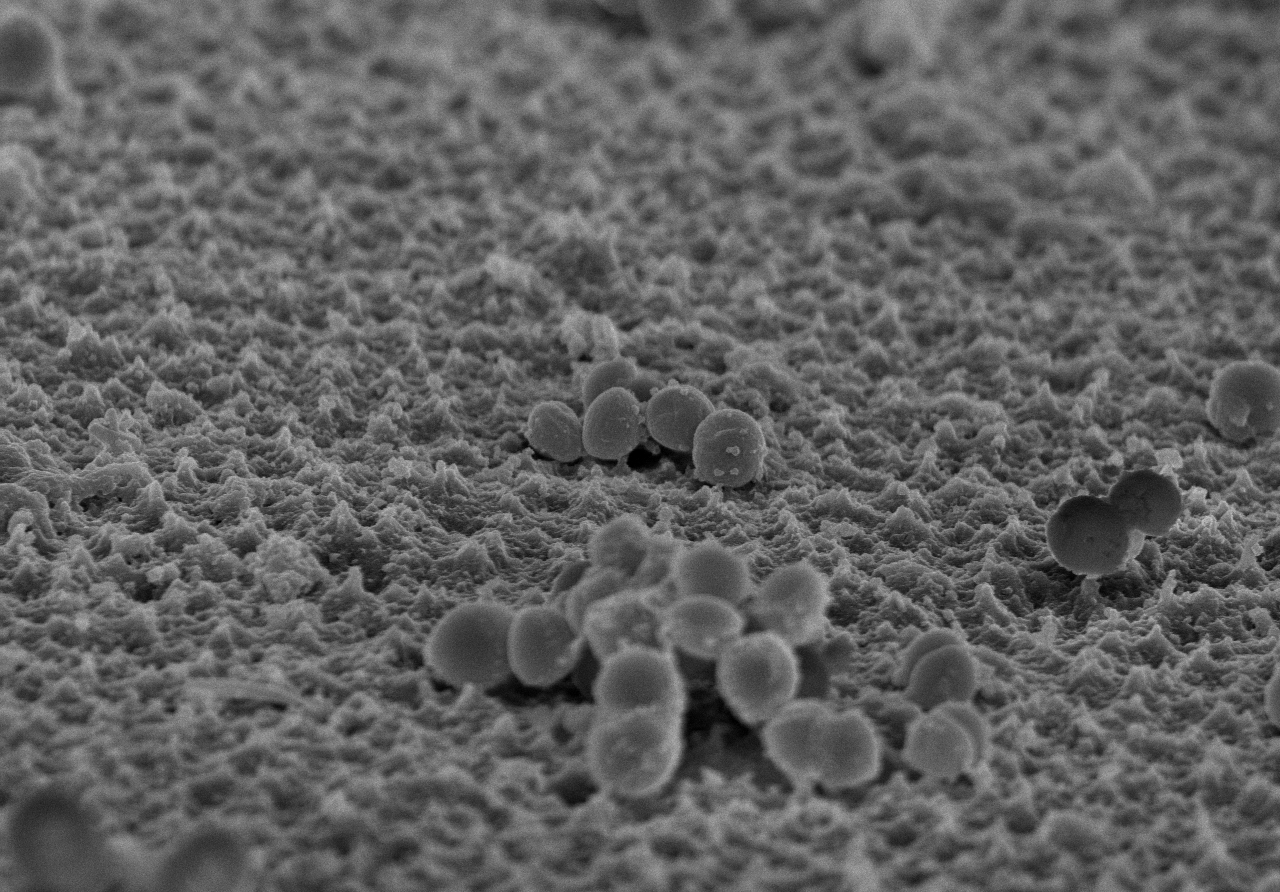
Novel conductive material towards fabrication of anti-biofouling implantable sensors and actuators
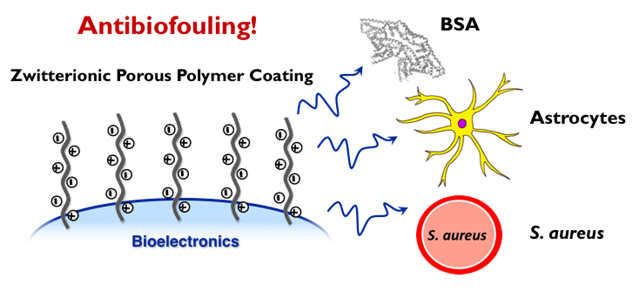
Neural interface
Development of fractal-based neurostimulation micro electrodes
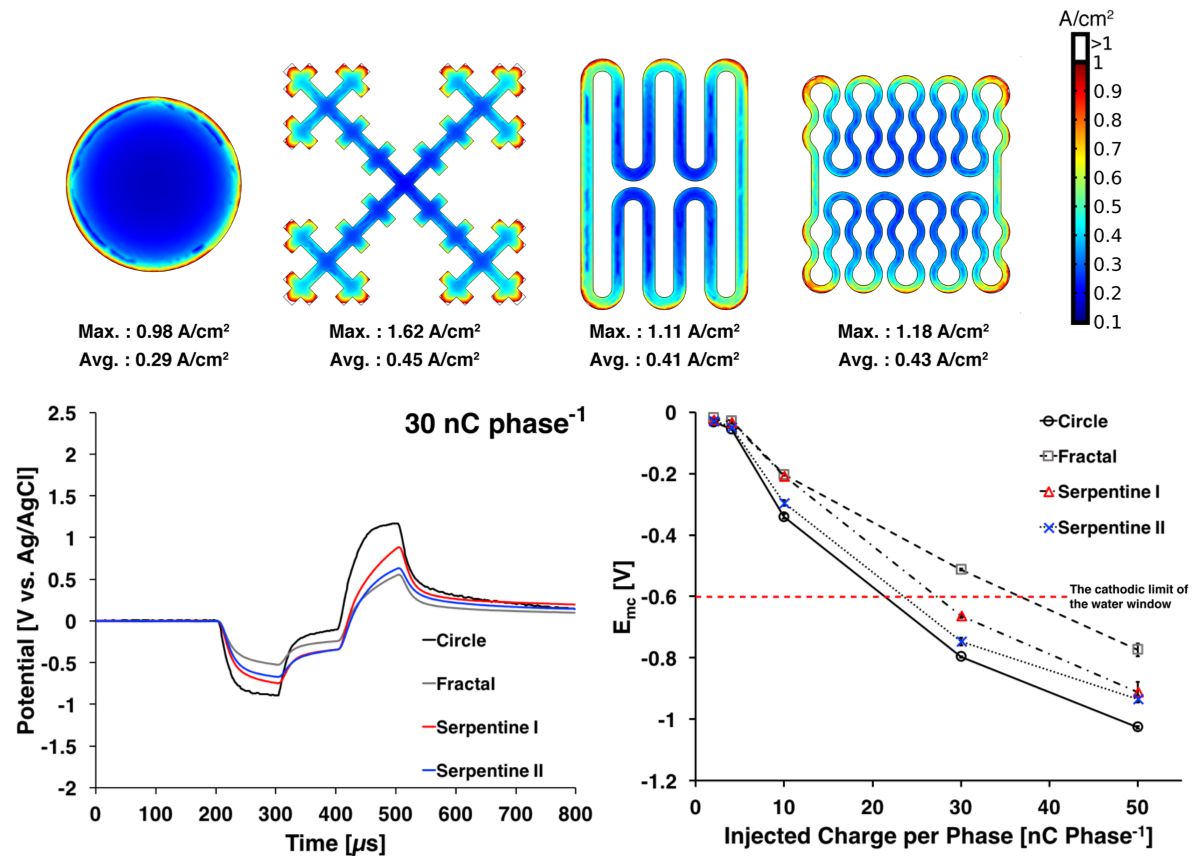
Prevention of Pt-corrosion of micro electrodes using a graphene monolayer
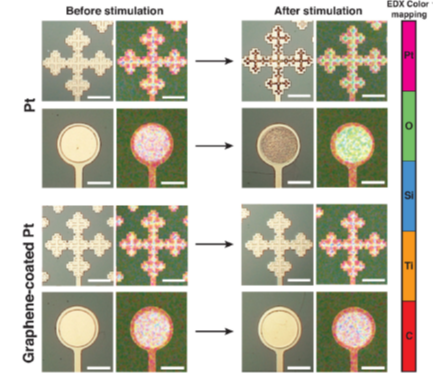
In vivo evaluation of novel neural interfaces
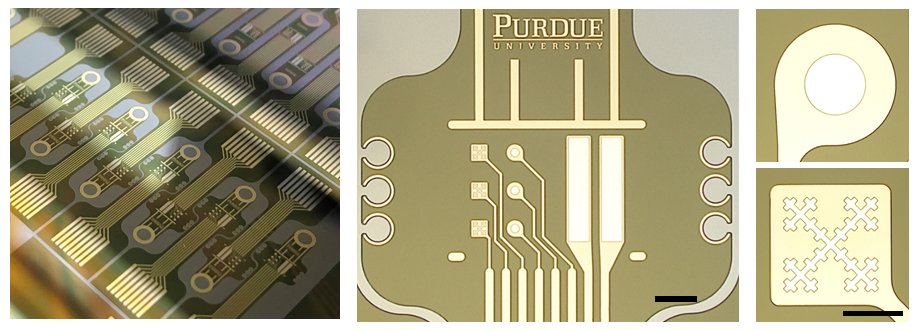
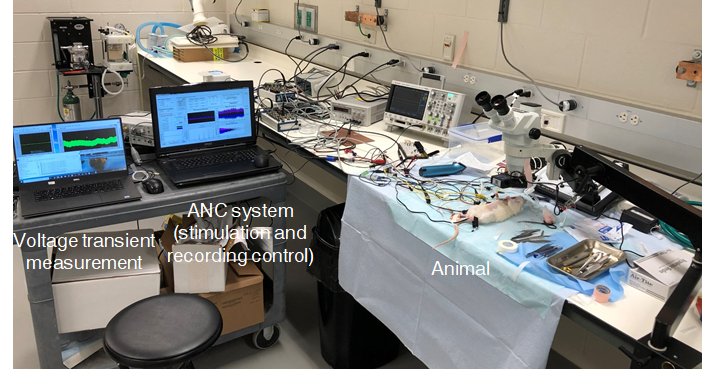